Fig. 16.1
This figure shows a complex, stellate hepatic fracture within the interlobar plane between the gallbladder bed and inferior vena cava in a high-fidelity surgical simulator. Hepatic venous bleeding is evident from the depths of the fracture. Modern technology makes it possible to create not only this wound but a wide variety of hepatic injury patterns within artificial tissues (Image courtesy of Operative Experience Inc.)
Because of the massive disruption of the hepatic parenchyma and capsule, spontaneous containment of hemorrhage is impossible in the type of wound depicted in Fig. 16.1. Patients with this pattern of injury often present in hemorrhagic shock. A positive FAST exam or peritoneal tap results in rapid transport of the patient to the operating room for exploratory laparotomy and continued resuscitation.
We propose that a comprehensive curriculum, based on this high-fidelity physical model/surgical simulator, could be developed to teach the operative management of severe hepatic trauma. Such a curriculum might include training in maneuvers such as manual compression of the liver; mobilization of the liver; perihepatic packing; Pringle maneuver; control of accessible, intrahepatic artery branches using clips or ligatures; mobilization of the omentum; omental packing of the liver; and placement of deep liver sutures. These maneuvers which are rarely performed in clinical practice are fundamental to the management of a complex liver injury. The simulator permits not only the demonstration but also the repeated and systematic practice of all these maneuvers, using standard surgical instruments. Additionally, the standardized nature of the model would allow for rigorous assessment of trainees to ensure correct performance.
The physical model takes into account the fact that the liver has a somewhat pyramidal shape that makes manual compression of the very thick, deep central zone difficult. By constructing the simulated liver in a three-dimensional anatomically correct fashion, it is possible to fold down the left lateral segment, as shown in Fig. 16.2. This allows the liver to assume a more globular shape that facilitates manual compression of the deep portions of the liver where the largest segments of the intraparenchymal hepatic veins reside. Compression of this deep posterior central zone of the liver is essential to immediate hemorrhage control because hepatic vein bleeding is not controlled by a Pringle maneuver. Mobilization of the left lateral segment can then be achieved by taking down the left triangular ligament in a way that does not involve a major entry into the bare area of the liver.
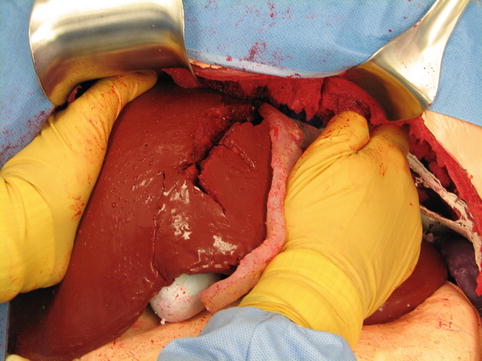
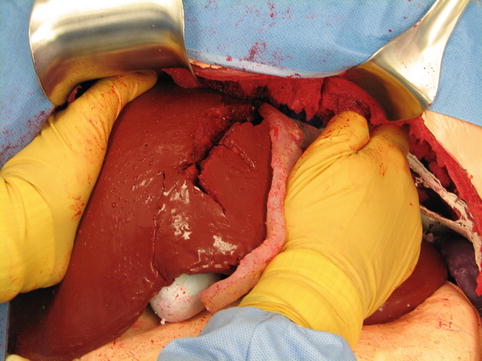
Fig. 16.2
A demonstration of manual compression of the liver after mobilization of the left triangular and falciform ligaments. The anterior body wall of the simulator has been removed to facilitate photography (Image courtesy of Operative Experience Inc.)
The photograph of Fig. 16.2 shows the cut left triangular and falciform ligaments. The falciform ligament has been divided all the way back to the vena cava within the areolar tissue of the bare area. This is potentially a tactical error, leading to bad outcomes. The injury pattern shown on the simulator carries a risk of associated injury to the retrohepatic vena cava or the extraparenchymal hepatic veins. In such a case, hepatic mobilization that destroys the suspensory ligaments of the liver, exposing the bare area, is extremely dangerous because it may release uncontrollable hemorrhage from injured retrohepatic, major veins.
Wide hepatic mobilization has long been recommended by most experts as an early, and almost automatic, step in the management of liver injuries. In most cases, it facilitates exposure and is safe. However, an exception to this rule exists in patterns of injury that might be associated with retrohepatic venous injuries. Mobilization of the liver should be a rational rather than an automatic decision. In our opinion, mobilization should be limited to the left triangular ligament and the falciform ligament anterior to the coronary ligaments, if there is a possibility that a spontaneously contained retrohepatic venous injury may exist.
Taking down the hepatic ligaments is easy to do but impossible to undo. Destruction of the spontaneous containment of a retrohepatic venous injury by surgical division of the coronary and triangular ligaments may leave the operator with no choice but to attempt a direct repair of the venous injury, using an atriocaval shunt, venovenous bypass, or some other heroic and high-risk approach. This potential catastrophe is largely avoidable if the operator is circumspect about hepatic mobilization in cases in which a contained retrohepatic/bare area hemorrhage may exist. In such cases, if the hepatic suspensory ligaments are damaged with leakage of blood or if injudicious hepatic mobilization has already begun when the blood is encountered, the suspensory ligaments should be repaired or reinforced to restore bare area containment.
Hepatic inflow occlusion by the Pringle maneuver is, together with manual compression and packing, one of the key initial maneuvers for hepatic hemorrhage control. Although almost any type of vascular clamp or a Rumel tourniquet could be used, we have found that a highly angled vascular clamp such as that shown in Fig. 16.3 is ideal. The clamp may be rapidly placed if the operator places the index finger of the left hand through the foramen of Winslow, loops the finger around the portal structures, and passes the clamp from a medial to lateral direction against the finger through the lesser omentum as shown in Fig. 16.3. With this direction of clamping, the handle of the clamp lies on the anterior stomach and remains out of the way during the rest of the operation.
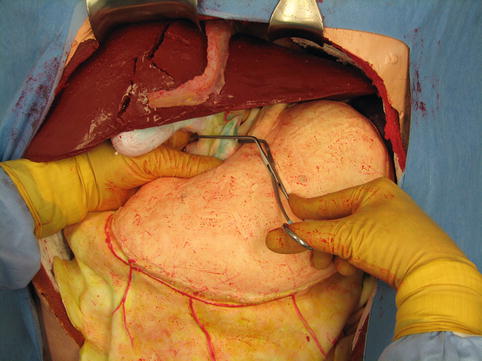
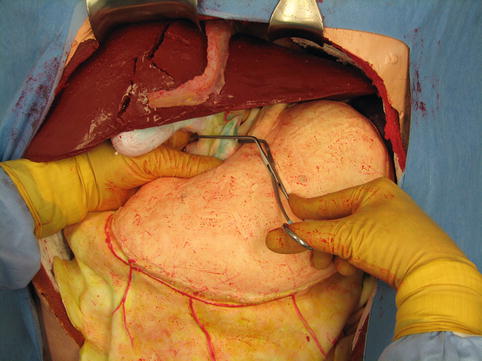
Fig. 16.3
A demonstration of the Pringle maneuver: with a finger in the foramen of Winslow, an angled vascular clamp is placed across the hepatic inflow vessels (Image courtesy of Operative Experience Inc.)
Effective hepatic inflow occlusion abolishes arterial and portal perfusion of the liver, except in the case of an aberrant left hepatic artery. The persistence of dark bleeding through or around the liver after hepatic inflow occlusion indicates hepatic venous injury. Bleeding through the fractured liver indicates an injury of the intraparenchymal portion of the hepatic veins. Dark blood issuing through the suspensory ligaments of the liver indicates potential extraparenchymal hepatic venous or retrohepatic vena cava injury.
Figure 16.4 shows the appearance of a rolled gauze pack. Over the years, one of the authors (RFB) has found multiple uses for gauze packs made in this way, particularly as an adjunct to manual pressure on bleeding sites, including injuries of the inferior vena cava. They also work well as rapidly placed tampons in large soft tissue wounds of the abdominal and flank muscles while attention is directed to more pressing injuries. In liver trauma, they are useful for perihepatic and subhepatic packing because they maintain their structure and can be placed and stacked in precise locations, for example, along the dome of the liver or against the hepatic suspensory ligaments.
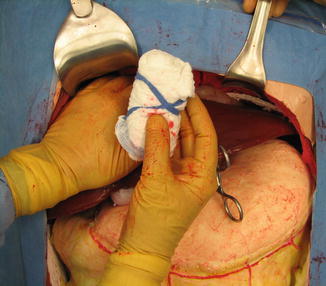
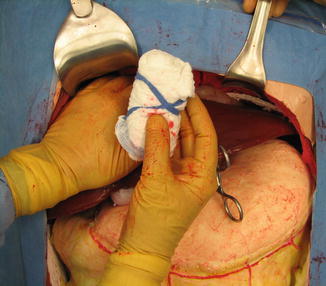
Fig. 16.4
The use of rolled gauze packs allows the application of focal pressure at selected points on the liver. The packs are created by folding standard laparotomy pads in thirds and rolling them into tight cylinders with the ribbon maintaining the shape. They are often used in combination with loose gauze packing (Image courtesy of Operative Experience Inc.)
As is true for many things in operative surgery, there has been no trial comparing the efficacy of packs made in this way to standard, loose gauze packing with regard to the control of bleeding. If the packs are premade and stored in sterile bags, dozens of them can be made available at the beginning of a case, which allows for extremely rapid abdominal packing, including the use of stacked rolls at actively bleeding sites.
High-energy transfer injuries of the liver, whether due to blunt bursting fractures or high-energy gunshot wounds, create extremely complex wounding patterns within the parenchyma. The area of destruction often contains, in addition to the primary laceration, multiple secondary and tertiary crevices, crags, and overhangs. Tissue within the wounded parenchyma is extremely friable. In the absence of major hemorrhage from hepatic vein injury, an effective Pringle maneuver permits entry into the injury site where accessible arterial and portal vein branches may be directly controlled with hemostatic surgical clips. This approach is illustrated in Fig. 16.5. High-pressure bleeding from arteries is the real target. The lesser branches of the portal vein and intraparenchymal hepatic veins which are low pressure vessels can be controlled by omental packing, deep hepatic sutures, and perihepatic gauze packing.
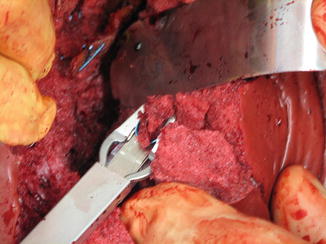
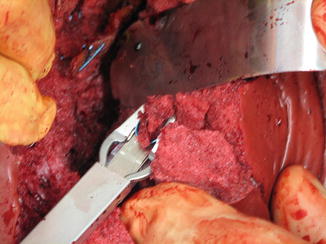
Fig. 16.5
After an effective Pringle maneuver, surgical clips can be used to control accessible bleeding vessels and bile ducts within the hepatic injury zone. Because the disrupted tissue is very friable, the use of suture ligatures is slower and generally less satisfactory than the use of the clips. Even the clips are not absolutely secure. One should be careful not to displace them during subsequent omental packing of the liver (Image courtesy of Operative Experience Inc.)
It is extremely difficult if not impossible to completely control all bleeding sites within complex burst fractures of the liver using direct measures such as surgical clips. Intraparenchymal packing with gauze is not widely used although the long-standing prejudice against this practice, based on clinical experiences, does not appear to have a strong scientific foundation. The best expedient for packing within the substance of the liver is the omental pack based on the right gastroepiploic artery.
In severe hepatic fractures, that is, those in which intraparenchymal hemostatic packing may be contemplated, the structural integrity of the omentum should be preserved during initial exploration of the lesser sac. Rather than clamping across the omentum to gain access to the lesser sac, the slightly more time-consuming expedient of mobilizing the omentum from the transverse colon in the avascular plane preserves the entire omentum for later use. The thickness of the omentum varies widely from individual to individual; in obese individuals it may be very substantial, whereas in lean young males, it may have relatively little fat.
The ability to demonstrate on the model the procedure for mobilizing the omentum on the right gastroepiploic artery and using it to pack a major liver defect is shown in Figs. 16.6, 16.7, 16.8, 16.9, 16.10, and 16.11. In nearly all patients no matter how obese, there is a thin area of the greater omentum below the left inferior aspect of the greater curvature of the stomach. This area is easily penetrated with finger dissection, which is the first step in mobilization. It is desirable to start the mobilization of the omentum in this location, just inferior to the short gastric arteries, because it makes virtually the entire omentum available for later use (Fig. 16.6).
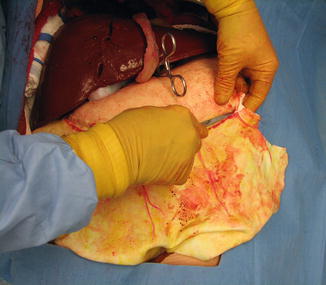
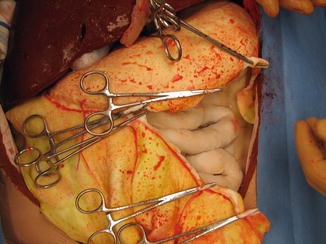
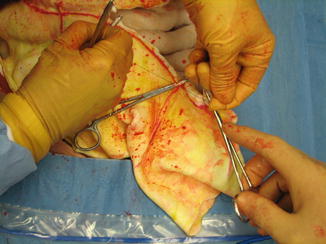
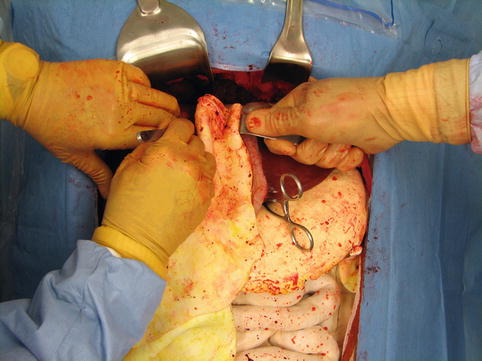
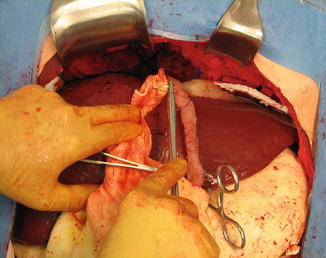
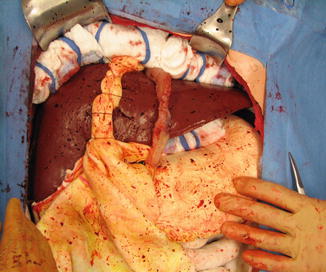
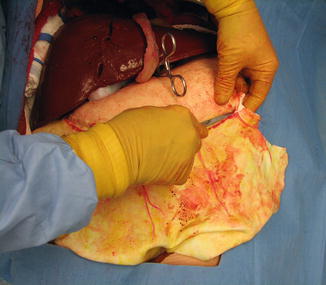
Fig. 16.6
Beginning mobilization of the greater omentum along the curvature of the stomach. Finger dissection has opened a rent in the thinnest portion of the omentum. The gastric branches of the gastroepiploic artery are isolated and divided between clamps (Image courtesy of Operative Experience Inc.)
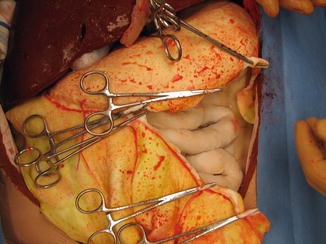
Fig. 16.7
Continued mobilization of the momentum from the greater curvature (Image courtesy of Operative Experience Inc.)
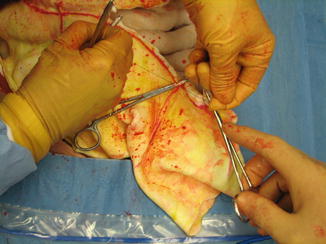
Fig. 16.8
Secure ligation of the branches of the gastroepiploic artery that will be packed into the liver is absolutely essential. The use of hemostatic clips is not recommended for this maneuver because the clips will be susceptible to displacement as the omentum is packed into the liver wound (Image courtesy of Operative Experience Inc.)
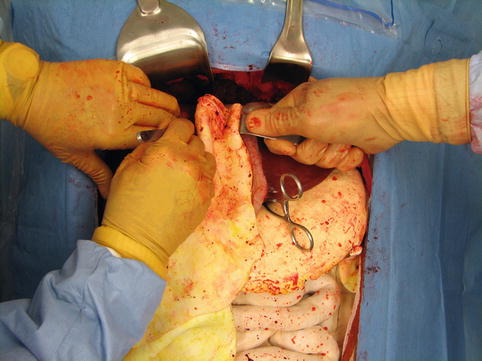
Fig. 16.9
The mobilized omentum is packed into liver injury with care to avoid a 360° twist of the pedicle or injury to the arteries within the omental pack (Image courtesy of Operative Experience Inc.)
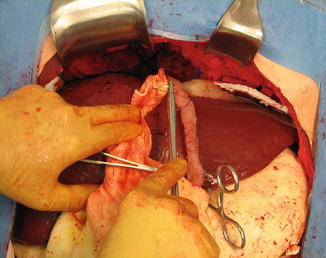
Fig. 16.10
The omental pack is secured within the liver with large sutures of number 1 Chromic on a blunt-tipped liver needle (Image courtesy of Operative Experience Inc.)
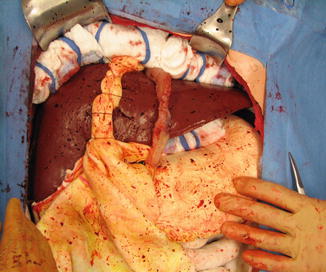
Fig. 16.11
Perihepatic packing using rolled gauze packs complements the hemostatic compression of the liver. The anterior body wall of the simulator has been removed to facilitate visualization. In clinical practice, packs would be inserted between the anterior surface of the liver and the diaphragm (Image courtesy of Operative Experience Inc.)
The dissection of the omentum is carried down to the greater curvature. The gastric branches of the gastroepiploic artery are now divided between clamps, along the wall of the stomach (Fig. 16.7). This process is repeated all along the greater curvature until a sufficient amount of omentum has been mobilized for the desired use.
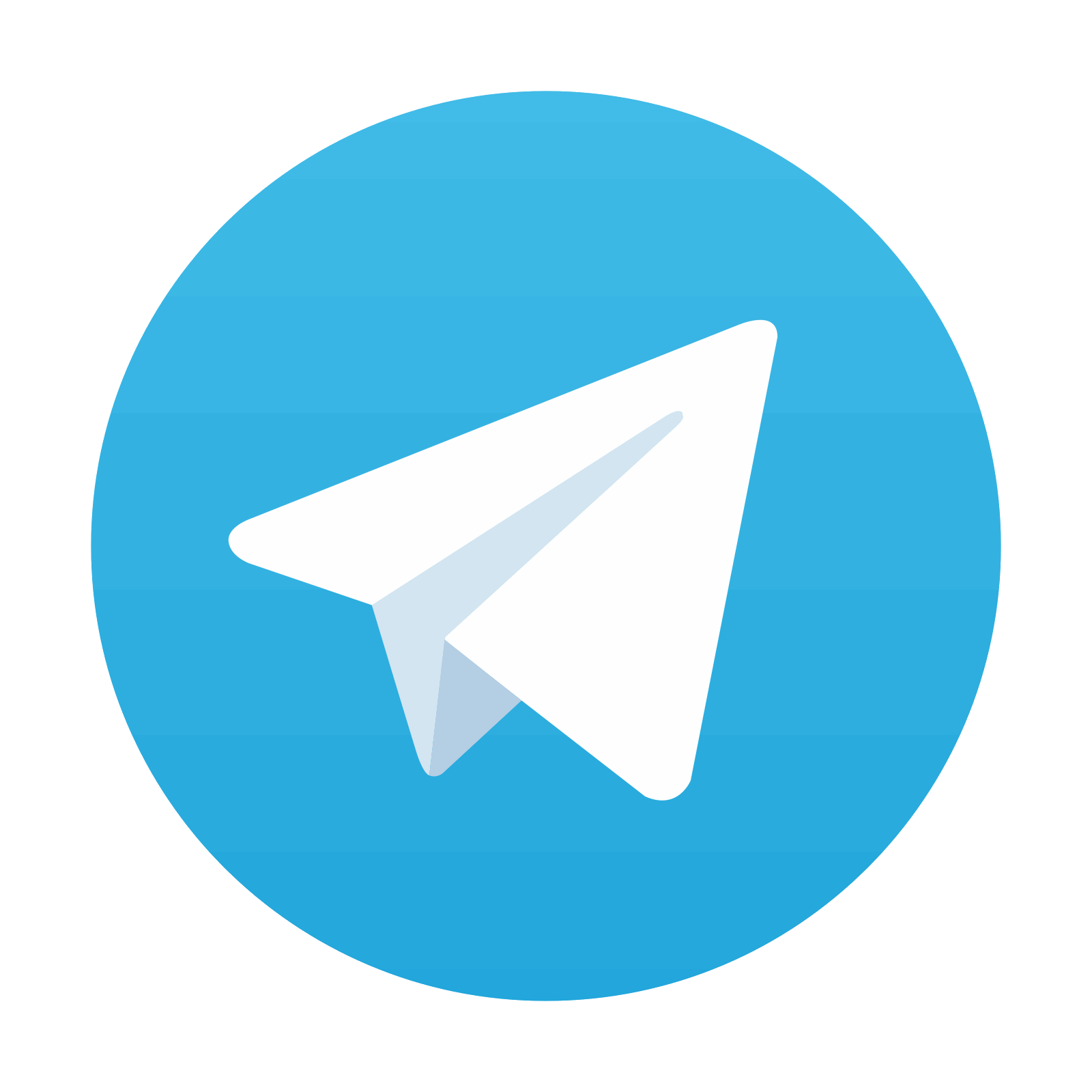
Stay updated, free articles. Join our Telegram channel

Full access? Get Clinical Tree
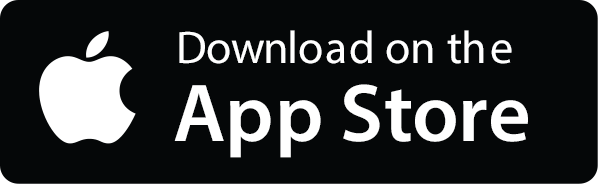
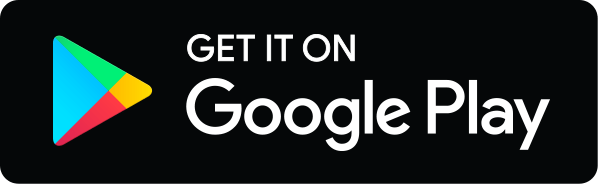