Keywords
LactateHyperlactatemiaTissue hypoxiaWarburg effectMetformin-associated lactic acidosis (MALA)Antiretroviral agentsLinezolidPropofol
Stereoisomers of lactate

Simplified diagram illustrating lactate formation from pyruvate occurring via glycolysis (cytoplasm) and oxidation of pyruvate to CO2 and water (mitochondria). LDH lactate dehydrogenase, ALT alanine aminotransferase
Approximately 20 mmol/kg/day of lactate is produced (~1300 mmol in a 70 kg man) in tissues such as skin (350 mmol), erythrocytes (302 mmol), brain (235 mmol), skeletal muscle (218 mmol), GI tract (115 mmol), and platelets and white blood cells (70 mmol). Lactate is utilized (metabolized) in the liver (722 mmol), kidney (386 mmol), heart (80 mmol), and other organs (102 mmol). Despite this high daily production of lactate, its circulating levels remain <1.5 mmol/L because of extensive utilization, as stated above. Lactic acidosis develops only when lactate production exceeds its utilization or when utilization of lactate is greatly reduced despite normal production, as in liver failure.
Lactic acidosis is a serious condition and carries increased morbidity and mortality. There is a linear relationship between lactate levels and mortality, and higher levels predict mortality. There are two types of lactic acidosis. The most common one is L-lactic acidosis, and less frequent one is D-lactic acidosis, and both types are presented below.
l-Lactic Acidosis
Production
As stated above, lactate is formed only from pyruvate (Fig. 5.2). This conversion is facilitated by lactate dehydrogenase (LDH). In this reaction, pyruvate is reduced to lactate, which requires oxidation of reduced nicotinamide adenine dinucleotide (NADH) to oxidized nicotinamide adenine dinucleotide (NAD+). NADH/NAD+ ratio determines the conversions between pyruvate and lactate. The normal lactate to pyruvate ratio is 10:1. As shown in the reaction below, lactate can be oxidized to pyruvate by LDH where NAD+ is reduced to NADH

- 1.
Increased glycolysis and pyruvate production, which are caused by intravenous (i.v.) glucose or epinephrine infusion, and metabolic or respiratory alkalosis. Alkalosis stimulates glycolysis by increasing the activity of a rate-limiting enzyme of glycolysis, phosphofructokinase (PFK). Acidosis and ATP inhibit PFK, leading to less lactate production
- 2.
An increase in NADH/NAD+ ratio due to hypoxic conditions
- 3.
Combination of above two processes
Hyperlactatemia Vs. Lactic Acidosis
Hyperlactatemia is defined as serum lactate levels of 2–5 mmol/L without acidosis. It usually occurs in conditions of adequate tissue perfusion and tissue buffering capacity. Hyperlactatemia is seen in conditions of activated aerobic glycolysis due to stress, elevated epinephrine levels with the use of β2-adrenergic agonists, asthma, alkalemia, and certain cytokine-stimulated glycolysis. Some drugs (antiretrovirals) may also cause hyperlactatemia. Serum [HCO3 −] may be slightly lowered by increased lactate levels, but the systemic pH may be minimally altered. Removal of offending agent or correction of clinical condition may improve hyperlactatemia.
In contrast to hyperlactatemia, lactic acidosis is seen in patients with global tissue hypoxia and is associated with severe consequences. Normalization of lactate levels is rather difficult (see Treatment).
Causes
- 1.
Generally, lactic acidosis is divided into two types: type A and type B acidosis
- 2.
Type A acidosis results from generalized or regional tissue hypoxia
- 3.
Type B acidosis results from biochemical abnormalities due to systemic diseases or toxins. Based on the underlying etiology, some authorities may divide type B lactic acidosis into type B1 (systemic diseases), type B2 (drugs and toxins), and type B3 (inherited)
- 4.
Note that both type A and type B conditions can be superimposed on one another rather commonly in any patient (metformin use in CHF patient)
Causes of type A lactic acidosis (clinical evidence of tissue hypoxia)
Cause | Mechanism(s) |
---|---|
Shock | |
Septic shock | Hypotension |
Hypovolemic shock | ↓ O2 delivery, ↑ glycolysis, ↓ ATP, ↑ pyruvate production→ ↑ lactic acid production |
Cardiogenic shock | ↓ O2 delivery, ↑ glycolysis, ↓ ATP, ↑ pyruvate production→ ↑ lactic acid production |
Hemorrhagic shock | ↓ O2 delivery, ↑ glycolysis, ↓ ATP, ↑ pyruvate production→ ↑ lactic acid production |
Severe tissue hypoxia | ↓ O2 delivery, ↑ glycolysis, ↓ ATP, ↑ pyruvate production→ ↑ lactic acid production |
Severe regional hypoperfusion due to hypotension | ↓ O2 delivery, ↑ glycolysis, ↓ ATP, ↑ pyruvate production→ ↑ lactic acid production |
Severe anemia (<4.0 g/dL) | Tissue hypoxia |
Severe asthma | Respiratory alkalosis stimulation of glycolysis and lactate production, tissue hypoxia, β-adrenergic stimulation, and lactate production |
Carbon monoxide poisoning | Carbon monoxide binds more avidly to Hgb than O2, leading to less delivery to tissues and hypoxia, inhibition of electron transport system, ↓ ATP, ↑ anaerobic glycolysis |
Causes of type B lactic acidosis (no clinical evidence of tissue hypoxia)
Causes | Mechanism(s) |
---|---|
Systemic disease (B1) | |
Liver disease | ↓ Lactate metabolism, ↓ pyruvate dehydrogenase complex (PDC)a activity, respiratory alkalosis, and hypoglycemia may precipitate lactate production |
Diabetes mellitus | Presence of microvascular disease and atherosclerosis compromising circulation, drug use such as metformin, ↓ PDC activity |
Renal failure and renal replacement therapies | Stimulation of lactate production by alkalinization due to HCO3 −, dialysate baths containing lactate |
Malignancy | Lymphomas, leukemias, and carcinomas (breast, lung, colon, pancreas), production of lactate by tumor cells via ↑ anaerobic glycolysis, ↑ cytokine production, hypoxia-inducible factor |
ATP depletion | ↑ Anaerobic glycolysis |
Thiamine deficiency | Inhibits PDC activity, thereby limiting glucose metabolism to glycolysis only |
Seizures | Increased muscle activity, compromised blood flow, and tissue hypoxia |
Hypoglycemia | Inhibits lactate uptake by liver, ↑ epinephrine release causing increased production of pyruvate |
Drugs/toxins (B2) | |
Metformin | Promotes lactate production from glucose in small intestine, ↑ NADH/NAD+ ratio, inhibits gluconeogenesis from lactate, inhibition of mitochondrial oxidative phosphorylation, patients with renal, hepatic, and cardiac failure are at risk |
Ethanol | Impairs gluconeogenesis from lactate to glucose, depletes NAD+ favoring lactate accumulation |
Methanol | Toxic products of methanol (formaldehyde, formic acid) inhibits oxidative phosphorylation and ATP synthesis |
Ethylene glycol | ↑ NADH/NAD+ ratio during metabolism of ethylene glycol via alcohol dehydrogenase |
Propylene glycol | Used as solvent (during infusion of lorazepam, nitroglycerine, or topical application of silver sulfadiazine), produces lactate during its metabolism via alcohol dehydrogenase. This reaction produces high NADH/NAD+ ratio |
Salicylates | Respiratory alkalosis-stimulated lactate production, inhibition of oxidative phosphorylation |
Cyanide poisoning | Inhibition of oxidative phosphorylation, ↓ ATP production, ↑ glycolysis, ↑ NADH/NAD+ ratio, leading to pyruvate conversion to lactate production |
Catecholamines | Epinephrine increases glycolysis and inhibits pyruvate formation from lactate. Increased vasoconstriction of skin, skeletal muscle, and splanchnic circulation with high concentrations of epinephrine and norepinephrine. Lactic acidosis may be the initial finding in pheochromocytoma |
Cocaine | Increased vasoconstriction |
Antiretrovirals (didanosine, zidovudine, stavudine, tenofovir) | Inhibition of mitochondrial DNA synthesis, ↓ ATP production, ↑ glycolysis |
Linezolid | Mitochondrial toxicity |
Propofol | Sedative, increased production of lactate on high doses due to uncoupling of oxidative phosphorylation |
Hereditary or acquired enzyme defects (B3) | (see below) |
Selected Conditions of L-Lactic Acidosis
Tissue Hypoxia
The most important cause of type A lactic acidosis is tissue hypoxia, which may be due to decreased perfusion or decreased O2 content. Shock of any etiology leads to hypotension, leading to lactic acid production. Other causes of tissue hypoxia include severe anemia, severe asthma, and carbon monoxide poisoning.
Sepsis and septic shock are due to macro- and microcirculatory dysfunction with decreased O2 and nutrient delivery to the peripheral tissues. The resultant hypoxia causes inhibition of mitochondrial oxidative phosphorylation and reduction in ATP synthesis as well as an increase in NADH/NAD+ ratio. This causes high lactate production, which is considered a useful marker of tissue hypoxia and a plan for fluid resuscitation in sepsis and septic shock.
During sepsis, insulin resistance occurs and gluconeogenesis increases. Also, whole-body glucose uptake by noninsulin-mediated mechanisms occurs. Glucose uptake by tissues such as skeletal muscle is mediated by cytokines such as tumor necrosis factor α and interleukin 6. Insulin resistance, gluconeogenesis, and cytokine stimulation of glucose uptake promote glycolysis and production of lactate from pyruvate.
Although elevated levels of lactate from anaerobic glycolysis due to tissue hypoxia have been reported to carry poor prognosis in sepsis, it was proposed that hyperlactatemia may also be caused by aerobic (not related to hypoxia) glycolysis. For example, sepsis causes high circulating levels of epinephrine, which stimulates Na/K-ATPase of skeletal muscle membrane and causes increased aerobic glycolysis. Thus, a major portion of the increased lactate levels may be attributed to aerobic glycolysis. This suggests that lactate is an unreliable marker of sepsis. Despite this critique, lactate is simple to measure, and its increasing levels during uncontrolled sepsis and decreasing levels to antibiotic therapy make it a dependable marker for sepsis.
Malignancy
In 1923, Otto Warburg demonstrated that cancer cells have the ability to increase their glycolytic activity and generate excessive production of lactic acid in the presence of O2. This phenomenon of aerobic glycolysis was subsequently named “Warburg effect.” Otto Warburg also observed that glucose uptake by tumor cells was much higher than normal cells and tumor cells metabolized more glucose to lactate. Indeed it was found that lactate levels were much higher in blood vessels leaving the tumor cells than blood vessels entering the tumor cells. Later, it was observed that hematologic malignancies such as lymphomas and leukemias generate very high levels of lactic acid. Patients with very high levels of lactic acid had poor prognosis. Other malignancies, including melanomas, multiple myeloma, lung cancer, sarcomas, breast cancer, as well as others, were also found to generate and release lactic acid into the surrounding environment.
- 1.
Glucose uptake by cancer cells is enhanced. Insulin-like growth factor-1 and tumor necrosis factor α, which are increased by cancer cells, stimulate glucose uptake
- 2.
Hexokinase and other glycolytic enzymes are overexpressed in many tumors
- 3.
Expression of transcription factors such as hypoxia-inducible transcription factor-1 (HIF-1) and c-Myc is enhanced in several cancer tissues. HIF-1 activates the genes encoding glycolytic enzymes, glucose, and lactate transporters, leading to enhanced glycolysis and lactic acid production
- 4.
HIF-1 indirectly inhibits pyruvate dehydrogenase (PDH), which converts pyruvate to acetyl-CoA for mitochondrial oxidation, thereby driving the accumulated pyruvate to lactate
- 5.
Thiamine deficiency caused by tumor cells and chemotherapy (methotrexate). Thiamine is a cofactor for PDH, and its deficiency causes decreased oxidative phosphorylation and energy (ATP) production
Lactic acid that is accumulated in cancer cells is transported from inside to outside by proton-linked monocarboxylate transporters (MCTs). Of the 14 MCT isoforms, only MCT1 and MCT4 are involved in lactic acid transport. Lactate, pyruvate, β-hydroxybutyrate, and acetoacetate are short-chain monocarboxylates. Once lactic acid is outside the cell, the pH of the extracellular microenvironment becomes severely acidic (in the range of 6.6), which promotes progression of tumor and imparts poor prognosis in several human cancers. MCT1 and MCT4 stimulate glycolysis in cancer cells in addition to their participation in lactic acid transport. Thus, inhibitors of MCT1 and MCT4 may decrease aerobic glycolytic pathway and lower lactate production. In addition to high activity of MCTs, increased activity of lactate dehydrogenase that converts pyruvate to lactate has been reported in cancer cells.
Treatment as well as prevention of malignancy-induced excess lactic acid is currently limited. Thiamine administration may be helpful. Also, inhibitors of lactate dehydrogenase and MCTs, once available, may play a significant role in preventing excess production of lactic acid in various human cancers.
Metformin-Associated Lactic Acidosis (MALA)
Metformin is a biguanide and is the recommended choice of oral hypoglycemic agent for patients with type 2 diabetes. Despite its widespread use, the occurrence of severe or fatal lactic acidosis is rather uncommon. The incidence of lactic acidosis at therapeutic levels of metformin is 3–5 cases per 100,000 patient years. Metformin lowers glucose by inhibition of hepatic gluconeogenesis and promoting glucose uptake by skeletal muscle. Additional mechanisms include interaction with the incretin axis through the action of glucagon-like peptide 1 and also antagonizing the actions of glucagon. Metformin is considered safe if used properly. Most of the drug is metabolized by the kidney with partial fecal elimination. Therefore, the dose requirements in patients with severe renal failure are different compared to a patient with normal renal function.
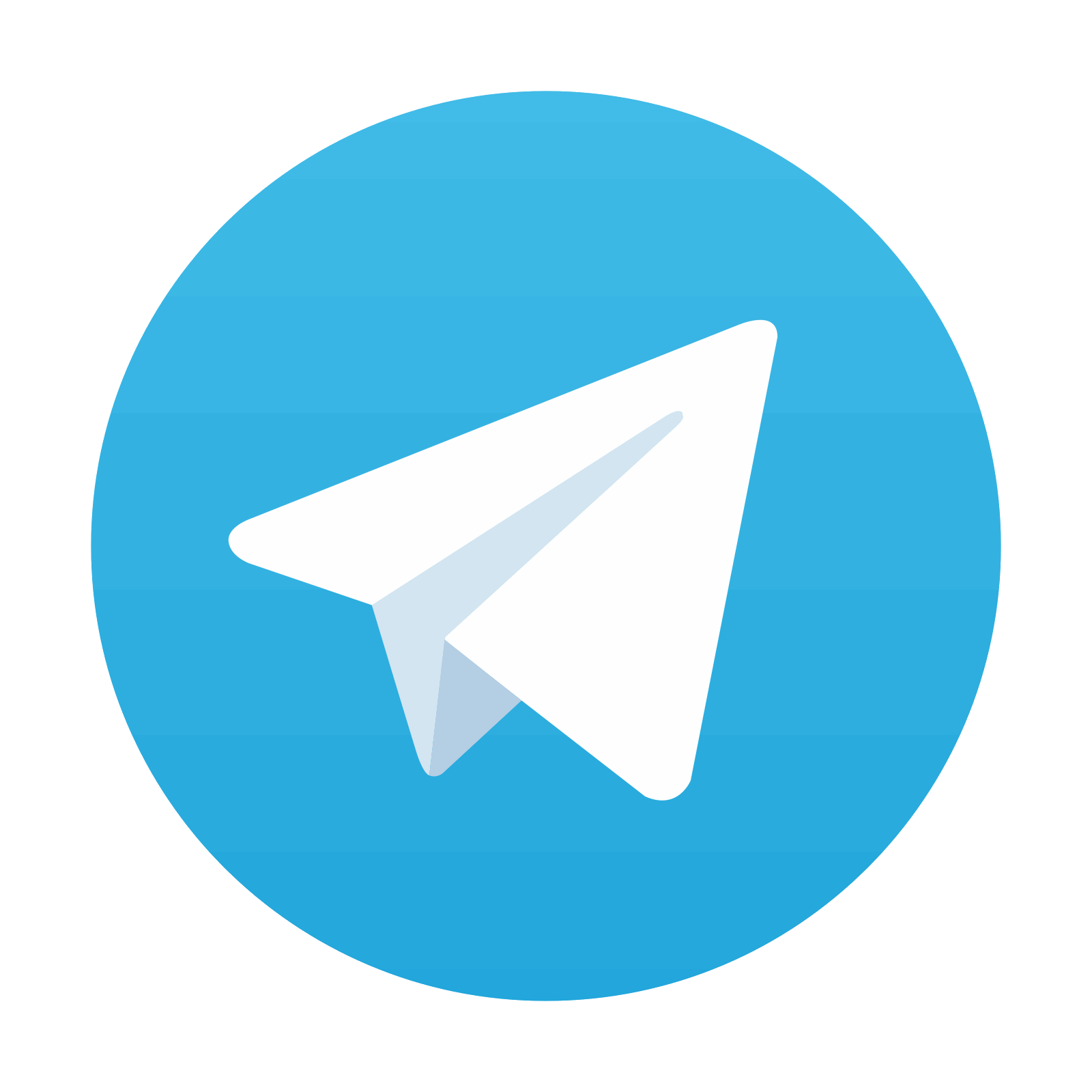
Stay updated, free articles. Join our Telegram channel

Full access? Get Clinical Tree
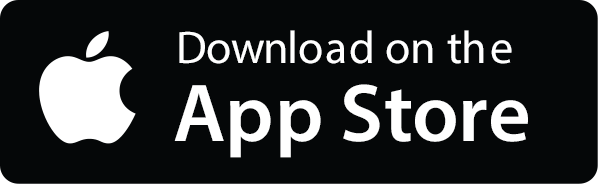
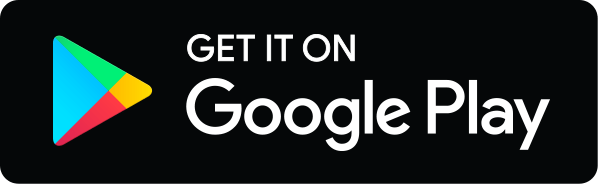