Keywords
CO2 ChemoreceptorsMedulla and ponsRespiratory musclesRespiratory acidosisAlveolar ventilationAcute respiratory acidosisChronic respiratory acidosisPhysiology
As stated in Chap. 4, respiratory acid–base disorders are due to changes in pCO2. In normal individuals, the arterial partial pressure of carbon dioxide (pCO2) is maintained at approximately 40 mmHg. This consistency of pCO2 is maintained by the alveolar ventilation. Lungs are the only organs that eliminate (excrete) CO2. Several physiologic mechanisms participate in the maintenance of CO2 balance (given later). Disturbance in any one of these mechanisms leads either to retention (hypercapnia or an increase in pCO2) or excessive elimination (hypocapnia or a decrease in pCO2) of CO2. The respiratory acid–base disorder that is associated with hypercapnia is called respiratory acidosis, whereas that associated with hypocapnia is known as respiratory alkalosis.
- 1.
CO2 production
- 2.
CO2 transport
- 3.
CO2 elimination
- 4.
Control of ventilation by central nervous system (CNS)
CO2 Production
CO2 is produced by metabolism of carbohydrates and fats.
Approximately 15000 mmol of CO2 are produced daily.
Heavy exercise generates severalfold higher quantity of CO2 than when the body is in resting state.
Lungs are the only organs that excrete all the CO2 that is produced.
Thus, the arterial pCO2 tension represents the balance between its production and elimination. The inspired CO2 is negligible.
CO2 Transport
The CO2 that is produced during metabolism is transported by the blood (plasma and red blood cells) to the lungs via pulmonary arteries.
The pCO2 difference (gradient) between the tissues and alveoli is only 6 mmHg.
In red blood cells, CO2 is converted to carbonic acid (H2CO3) in the presence of carbonic anhydrase.
H2CO3 then dissociates into H+ and HCO3 −. It is this HCO3 − that diffuses into the plasma via Cl/HCO3 exchanger and is carried to the lungs, where it is converted back to CO2.
CO2 Elimination
Alveolar ventilation is the major determinant of CO2 elimination.
A decrease in alveolar ventilation causes retention of CO2, resulting in an increase in total body CO2 balance.
Other determinants include blood flow through the aerated lung, diffusion of CO2 from the capillary to the alveolar space, and physiologic dead space.
CNS Control of Ventilation
- 1.
Sensors (chemoreceptors)
- 2.
Central controller (medulla and pons)
- 3.
Effectors (respiratory muscles)
Sensors
- 1.
Central chemoreceptors , located in the medulla, are surrounded by the interstitial and cerebrospinal fluid and respond to changes in [H+] or pCO2. For example, an increase in [H+], i.e., a decrease in pH, stimulates respiration and decreases pCO2. These responses, in turn, raise pH. On the other hand, a decrease in [H+] or an increase in pH depresses alveolar ventilation and causes retention of pCO2, so that the pH is returned to near normal. An increase in pCO2 stimulates ventilation, whereas a decrease depresses ventilation. Central chemoreceptors are not sensitive to changes in pO2
- 2.
Peripheral chemoreceptors are located in the carotid body and aortic arch. They respond to decreases in pO2 and pH and increases in pCO2. The sensitivity to changes in pO2 begins at around 500 mmHg, but little response occurs until pO2 is <60 mmHg. The receptors of carotid body are more sensitive to decrease in pO2 (hypoxemia) than the receptors located in the aortic arch
Medullary Center
- 1.
Central controller includes neurons located in the medulla and pons, which are referred to as respiratory centers
- 2.
Medullary respiratory center has two identifiable areas of neurons. One group of neurons is located in the dorsal region of the medulla (nucleus tractus solitarius). It is called the dorsal respiratory group, which is involved in inspiration (firing on inspiration). In addition, these neurons receive afferent stimuli from peripheral and central chemoreceptors and from receptors in the lung. The other group of neurons, the ventral respiratory group, controls both expiration (firing on expiration) and inspiration
Effectors
- 1.
Once changes are sensed in the medullary respiratory center, they are transmitted through nerves to the muscles of respiration (diaphragm, intercostal muscles, abdominal muscles, and sternocleidomastoid muscles). It is the coordinated effort of these muscles that is responsible for ventilation
Any disturbance in sensing and signaling results in either hypercapnia or hypocapnia, leading to either respiratory acidosis or respiratory alkalosis, respectively. Let us discuss respiratory acidosis.
Respiratory Acidosis
Respiratory acidosis, also called primary hypercapnia , is initiated by an increase in arterial pCO2. This increase is related to decreased excretion relative to the production of CO2. Whenever pCO2 increases, the pH falls. The excess H+ is immediately (within minutes) buffered by nonbicarbonate buffers, such as hemoglobin, phosphates, and plasma proteins, so that HCO3 − is not used up. During this buffering, some HCO3 − is also formed from dissociation of H2CO3. The acute nonbicarbonate buffer response is completed within 10–15 min, and a steady state condition persists for 1 h. If hypercapnia continues for >12 h, the kidneys generate additional HCO3 − by excretion of H+, and the maximum level of HCO3 − generation is completed within 3–5 days. Thus, respiratory acidosis can be classified into acute (<12 h) or chronic (>5 days) types. Both acute and chronic hypercapnias are associated with hypoxemia.
Pathophysiology
From the above discussion, respiratory acidosis can develop from any or combination of the following ventilation abnormalities: (1) overproduction of CO2; (2) decreased alveolar ventilation; (3) impaired gas exchange; (4) decreased respiratory center response; and (5) abnormalities of the chest wall and respiratory muscles.
Overproduction of CO 2
Clinical settings associated with CO2 production
Increase | Decrease |
---|---|
Fever High glucose loads Exercise Hyperthermia Hyperthyroidism Multiorgan failure Increased work of breathing (severe dyspnea) | Hypothermia High fat loads Inactivity (sleep) Hypothyroidism Weight loss Decreased work of breathing (mechanical ventilation, sedatives) |
Decreased Alveolar Ventilation (Hypoventilation)

Alveolar hypoventilation causes not only CO2 retention but also inadequate (poor) oxygenation. Impaired response of medullary CO2 receptors (due to narcotics) or carotid pO2 receptors (due to trauma or surgical removal) may cause alveolar hypoventilation.
Increase in V D (dead space) alone rarely causes alveolar hypoventilation unless the ratio of V D/V T (dead space to tidal volume) exceeds 0.6. Normal V D/V T ratio is 0.3. Conditions such as pulmonary embolism and shock lung may increase V D and result in high ratio. Also, relative decrease in V T can be seen in patients with increased respiratory rate (rapid but shallow breathing) due to neuromuscular disease and pulmonary edema or fibrosis. Increased V D/V T ratio is thought be the mechanism for acute exacerbation of COPD due to rapid shallow breathing. Thus, V D is an important contributor of alveolar hypoventilation.
Impaired Gas Exchange
Impaired alveolar gas exchange (ventilation–perfusion mismatch) can lead to hypoxemia and hypercapnia. Patients with lung disease and superimposed acute conditions such as pulmonary edema and pneumonia can cause impaired gas exchange with resultant hypoxemia and hypercapnia.
Decreased Respiratory Center Response
Drugs such as sedatives and narcotics, CNS diseases such as medullary infarcts and tumors, and diseases like hypothyroidism cause hypercapnia by inhibiting the central respiratory drive. Sedatives and narcotics should be used with caution in patients with severe asthma or COPD who retain CO2. However, some individuals with anxiety or pain who develop hypocapnia because of hyperventilation may benefit from the use of these drugs by lowering pH.
Abnormalities of the Chest Wall and Respiratory Muscles
Hypercapnia develops despite normal central drive and lung structure. Disorders of respiratory muscles and motor neurons are examples of this pathophysiologic mechanism of hypercapnia. Fatigue and weakness of diaphragm is an important contributor for hypercapnia. Electrolyte abnormalities such as severe hypokalemia and hypophosphatemia and malnutrition may cause respiratory muscle weakness and cause CO2 retention. Chest wall stiffness such as kyphoscoliosis is another cause for hypercapnia.
Secondary Physiologic Response to Hypercapnia
Acute respiratory acidosis: For each mmHg increase in pCO2, HCO3 − increases by 0.1 mEq/L.
Chronic respiratory acidosis: For each mmHg increase in pCO2, HCO3 − increases by 0.4 mEq/L.
Relationship between hypercapnia, secondary response, and pH
Type | pCO2 (mmHg) | Expected secondary response (compensation) | Expected serum [HCO3 −] | pH (calculated from Henderson equation) after compensation |
---|---|---|---|---|
Normal | 40 | – | 24 | 7.40 |
Acute | 70a | For each mmHg increase in pCO2, HCO3 − increases by 0.1 mEq/L, ΔpCO2 = 30 (70–40 = 30 × 0.1 = 3) | 27 (24 + 3 = 27) | 7.18 |
Chronic | 70a | For each mmHg increase in pCO2, HCO3 − increases by 0.4 mEq/L, ΔpCO2 = 30 (70–40 = 30 × 0.4 = 12) | 36 (24 + 12 = 36) | 7.34 |
Mechanism of Secondary Physiologic Response
Acute Respiratory Acidosis
An increase in pCO2 increases the production of carbonic acid (H2CO3) and H+ production. As a result of increased H+, the blood pH decreases (as shown in Table 12.2). The secondary physiologic response for this low pH or hypercapnia is an increase in plasma HCO3 − concentration, which is due to buffering of H+ by nonbicarbonate buffers such as red blood cells, hemoglobin, and tissue buffers. The increase in HCO3 − concentration is 0.1 mEq/L for each 1 mmHg increase in pCO2 above 40 mmHg (as shown in Table 12.2). The adaptive increase in HCO3 − concentration is complete in 10–15 min. Following this period of time, no further changes in HCO3– occur for hours, and this period is called acute steady state.
Chronic Respiratory Acidosis
As stated above, the kidney responds to chronic hypercapnia by increasing serum HCO3 − concentration. This occurs by increasing excretion of H+ mostly in the form of NH4 + (see Chap. 2). These changes start to happen 12–24 h after hypercapnia. Excretion of H+ results in formation and reabsorption of HCO3 −. This increase in HCO3 − is complete in 3–5 days. As shown in Table 12.2, for each mmHg increase in pCO2, HCO3 − increases by 0.4 mEq/L. Secretion of H+ and reabsorption of HCO3 − seem to occur in the proximal and distal tubules. Studies have shown an increased activity of luminal Na/H exchanger and basolateral Na/HCO3 cotransporter in the proximal tubule. Also, chronic hypercapnia has been shown to induce luminal insertion of H-ATPase in the proximal tubule and in type A intercalated cells of the cortical and medullary collecting ducts as well as abundance of basolateral Cl/HCO3 exchanger in the latter type of cells. These studies provide evidence for increased luminal H+ secretion and increased basolateral HCO3 − reabsorption in chronic hypercapnia. With the increase in serum HCO3 − concentration, a new steady develops, but the blood pH will not return to normal. Therefore, the patient will have persistent acidic pH despite high concentration of HCO3 −.
The increased HCO3 − reabsorption is associated with inhibition of Cl− reabsorption. As a result, Cl− is excreted in the urine with depletion of body stores. Cl− is also excreted with Na+ and K+. Thus, persistent hypochloremia exists in chronic respiratory acidosis.
Acute Respiratory Acidosis
Causes
Causes of acute respiratory acidosis
Depression of medullary respiratory center |
Drugs, anesthetics, sedatives, opiates |
Cerebral trauma or infarct |
Central sleep apnea |
Cardiac arrest |
Failure of motor functions of respiratory muscles and chest wall |
Drugs: succinylcholine, curare, aminoglycosides, fluoroquinolones |
High cervical cordotomy |
Myasthenia gravis crisis |
Guillain–Barrè syndrome |
Status epilepticus |
Tetanus |
Acute botulism poisoning |
Familial hypokalemic periodic paralysis |
Severe hypophosphatemia |
Airway obstruction |
Aspiration |
Laryngospasm |
Severe bronchospasm |
Obstructive sleep apnea |
Ventilatory defects |
Flail chest |
Pneumothorax |
Hydrothorax |
Adult respiratory distress syndrome |
Acute pulmonary embolism |
Acute pulmonary edema |
Severe asthma |
Severe pneumonia |
Mechanical ventilation, increased production of CO2 due to high carbohydrate feedings and fixed minute ventilation |
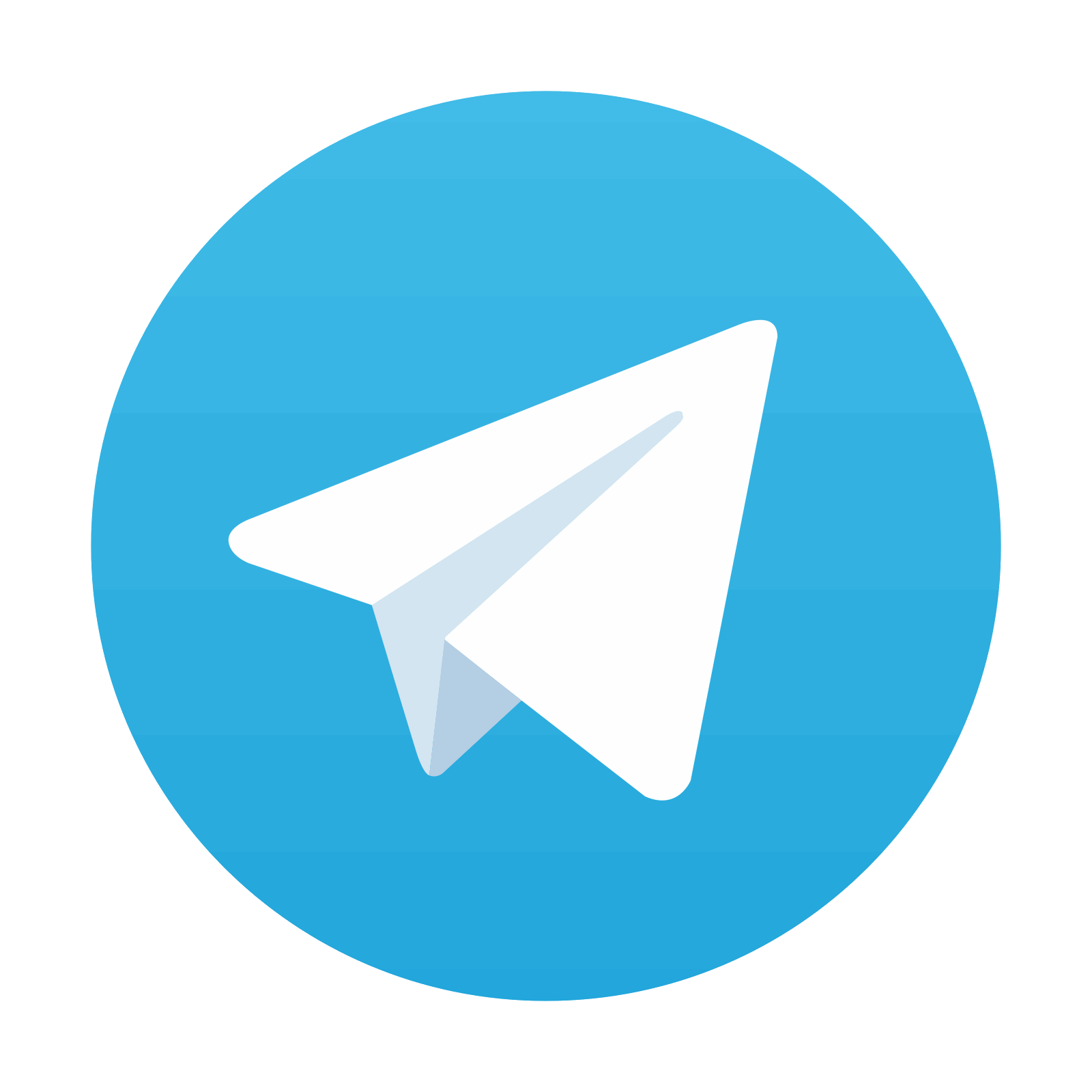
Stay updated, free articles. Join our Telegram channel

Full access? Get Clinical Tree
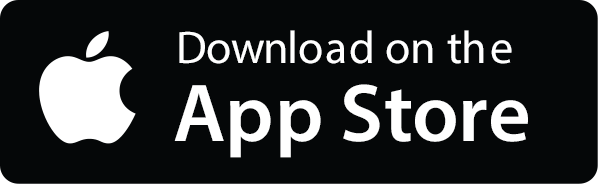
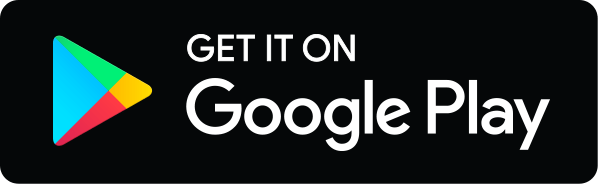