. Thus, pH is defined as the negative logarithm of the [H+]. An inverse relationship exists between pH and [H+]. In other words, as the pH increases, the [H+] decreases and vice versa. Cells cannot function at a pH below 6.8 and above 7.8. The normal arterial pH ranges from 7.38 to 7.42, which translates to a [H+] of 38–42 nmol/L. Blood pH is under constant threat by endogenous acid and base loads. If not removed, these loads can cause severe disturbances in blood pH, and thus impair cellular function. However, three important regulatory systems prevent changes in pH and thus maintain blood pH in the normal range. These protective systems, as previously stated, are buffers, lungs, and kidneys.
Keywords
Acid–base physiologyProduction of endogenous acidsProduction of endogenous basesMaintenance of normal pHUrinary acidificationAcid–base physiology deals with the maintenance of normal hydrogen ion concentration (abbreviated as [H+]) in body fluids. The normal [H+] in the extracellular fluid is about 40 nmol/L or 40 nEq/L (range 38–42 nmol/L), which is precisely regulated by an interplay between body buffers, lungs, and kidneys. Since many functions of the cell are dependent on the optimum [H+], it is extremely important to maintain [H+] in blood ~ 40 nmol/L. Any deviation from this [H+] results either in acidemia ([H+] > 40 nmol/L) or alkalemia ([H+] < 40 nmol/L). This chapter provides an overview of the role of buffers, lungs, and kidneys in regulating [H+] in body fluids. The [H+] in blood is so low that it is not measured routinely. However, the [H+] is measured as pH, which is expressed as:
![$$ \text{pH}=-\log \,[{{\text{H}}^{\text{+}}}]. $$](https://i0.wp.com/abdominalkey.com/wp-content/uploads/2017/06/A304669_1_En_26_Chapter_Equ1.gif?w=960)
(26.1)
Thus, pH is defined as the negative logarithm of the [H+]. An inverse relationship exists between pH and [H+]. In other words, as the pH increases, the [H+] decreases and vice versa. Cells cannot function at a pH below 6.8 and above 7.8. The normal arterial pH ranges from 7.38 to 7.42, which translates to a [H+] of 38–42 nmol/L.
Blood pH is under constant threat by endogenous acid and base loads. If not removed, these loads can cause severe disturbances in blood pH, and thus impair cellular function. However, three important regulatory systems prevent changes in pH and thus maintain blood pH in the normal range. These protective systems, as previously stated, are buffers, lungs, and kidneys.
Production of Endogenous Acids and Bases
An acid is a proton donor, whereas a base is a proton acceptor. Under physiological conditions, the diet is a major contributor to endogenous acid and base production .
Endogenous Acids
The oxidation of dietary carbohydrates, fats, and amino acids yields CO2. About 15,000 mmol of CO2 are produced by cellular metabolism daily. This CO2 combines with water in the blood to form carbonic acid (H2CO3):

(26.2)
This reaction is catalyzed by carbonic anhydrase (CA), an enzyme present in tissues and red blood cells but absent in plasma. When H2CO3 dissociates into CO2 and H2O (a process called dehydration), the CO2 is eliminated by the lungs. For this reason, H2CO3 is called a volatile acid .
In addition to volatile acid, the body also generates nonvolatile (fixed) acids from cellular metabolism. These nonvolatile acids are produced from sulfur-containing amino acids (i.e., cysteine and methionine) and phosphoproteins. The acids produced are sulfuric acid and phosphoric acid, respectively. Other sources of endogenous nonvolatile acids include glucose, which yields lactic and pyruvic acids; triglycerides, which yield acetoacetic and β-hydroxybutyric acids; and nucleoproteins, which yield uric acid. Hydrochloric acid is also formed from the metabolism of cationic amino acids (i.e., lysine, arginine, and histidine). Sulfuric acid accounts for 50 % of all acids produced. A typical North American diet produces 1 mmol/kg/day of endogenous nonvolatile acid.
Under certain conditions, acids are produced from sources other than the diet. For example, starvation produces ketoacids, which can accumulate in the blood. Similarly, strenuous exercise generates lactic acid. Drugs such as corticosteroids cause endogenous acid production by enhancing catabolism of muscle proteins .
Endogenous Bases
Endogenous base (HCO3 −) is generated from anionic amino acids (glutamate and aspartate) in the diet. Also, citrate or lactate generated during metabolism of carbohydrate yields HCO3 −. Vegetarian diets contain high amounts of anionic amino acids and small amounts of sulfur and phosphate-containing proteins. Therefore, these diets generate more base than acid. In general, the production of acid exceeds that of base in a person ingesting a typical North American diet .
Maintenance of Normal pH
Buffers
All acids that are produced must be removed from the body in order to maintain normal blood pH. Although the kidneys eliminate most of these acids, it takes hours to days to complete the process. Buffers (both cellular and extracellular) are the first line of defense against wide fluctuations in pH.
The most important buffer in blood is bicarbonate/carbon dioxide (HCO3 −/CO2). Other buffer systems are disodium phosphate/monosodium phosphate (Na2HPO4 2−/NaH2PO4 −), and plasma proteins. In addition, erythrocytes contain the important hemoglobin (Hb) system, reduced Hb (HHb−) and oxyhemoglobin (HbO2 2−). Bones also participate in buffering.
The HCO3 −/CO2 system provides the first line of defense in protecting pH. Its role as a buffer can be described by incorporating this system into the Henderson–Hasselbalch equation as follows:
![$$ \text{pH}=\text{pKa}+\log \,\frac{[\text{HCO}{{{}_{3}}^{-}}]}{[{{\text{H}}_{\text{2}}}\text{C}{{\text{O}}_{\text{3}}}]}. $$](https://i0.wp.com/abdominalkey.com/wp-content/uploads/2017/06/A304669_1_En_26_Chapter_Equ3.gif?w=960)
(26.3)
Although H2CO3 cannot be measured directly, its concentration can be estimated from the partial pressure of CO2 (pCO2) and the solubility coefficient (α) of CO2 at known temperature and pH. At normal temperature of 37 °C and pH of 7.4, the pCO2 is 40 mmHg, α is 0.03, and pKa is 6.1. The Henderson–Hasselbalch equation can be appropriately written as:
![$$ \text{pH}=6.1+\log \,\frac{[\text{HC}{{\text{O}}_{3}}^{-}]}{0.03\times \text{pC}{{\text{O}}_{\text{2}}}}. $$](https://i0.wp.com/abdominalkey.com/wp-content/uploads/2017/06/A304669_1_En_26_Chapter_Equ4.gif?w=960)
(26.4)
Normal plasma [HCO3 −] is 24 mEq/L. Therefore,

(26.5)
It should be noted from the Henderson–Hasselbalch equation that the pH of a solution is determined by the pKa and the ratio of [HCO3 −] to pCO2, and not by their absolute values. Thus, because the kidneys regulate the [HCO3 −] and the lungs pCO2, the kidneys and lungs determine the pH of extracellular fluids.
Phosphate buffers are effective in regulating intracellular pH more efficiently than extracellular pH. Their increased effectiveness intracellularly is due to their higher concentrations inside the cell. Also, the pKa of this system is 6.8, which is close to the intracellular pH .
Plasma proteins contain several ionizable groups in their amino acids that buffer either acids or bases. For example, the imidazole groups of histidine and the N-terminal amino groups have pKa that are close to extracellular pH and thus function as effective buffers. In blood, Hb is an important protein buffer because of its abundance in red blood cells.
Extracellular buffering to an acid load is complete within 30 min. Subsequent buffering occurs intracellularly and takes several hours to complete. Most of this intracellular buffering occurs in bone.
It is apparent from the Henderson–Hasselbalch Eq. 26.3 that any change either in [HCO3 −] or pCO2 can cause a change in blood pH. The acid–base disturbance that results from a change in plasma [HCO3 −] is termed a metabolic acid–base disorder , whereas that due to a change in pCO2 is called a respiratory acid–base disorder .
Lungs
After buffers, the lungs are the second line of defense against pH disturbance. In a normal individual, pCO2 is maintained around 40 mmHg. This pCO2 is achieved by expelling the CO2 that is produced by cellular metabolism through the lungs. Any disturbance in the elimination of CO2 may cause a change in blood pH. Thus, alveolar ventilation maintains normal pCO2 to prevent an acute change in pH. Alveolar ventilation is controlled by chemoreceptors located centrally in the medulla and peripherally in the carotid body and aortic arch. Blood [H+] and pCO2 are important regulators of alveolar ventilation. The chemoreceptors sense the changes in [H+] or pCO2 and alter alveolar ventilatory rate. For example, an increase in [H+], i.e., a decrease in pH, stimulates ventilatory rate and decreases pCO2. These responses, in turn, raise pH (see Eq. 26.4). Conversely, a decrease in [H+] or an increase in pH depresses alveolar ventilation and causes retention of pCO2 so that the pH is returned to near normal. An increase in pCO2 stimulates ventilatory rate, whereas a decrease depresses the ventilatory rate. The respiratory response to changes in [H+] takes several hours to complete .
Kidneys
As stated earlier, 1 mmol/kg/day of fixed acid is produced from the diet. If not removed, this acid is retained and plasma [HCO3 −] decreases. The result is metabolic acidosis. In a healthy individual, metabolic acidosis does not occur because the kidneys excrete the acid load and maintain plasma [HCO3 −] around 24 mEq/L. The maintenance of [HCO3 −] is achieved by three renal mechanisms :
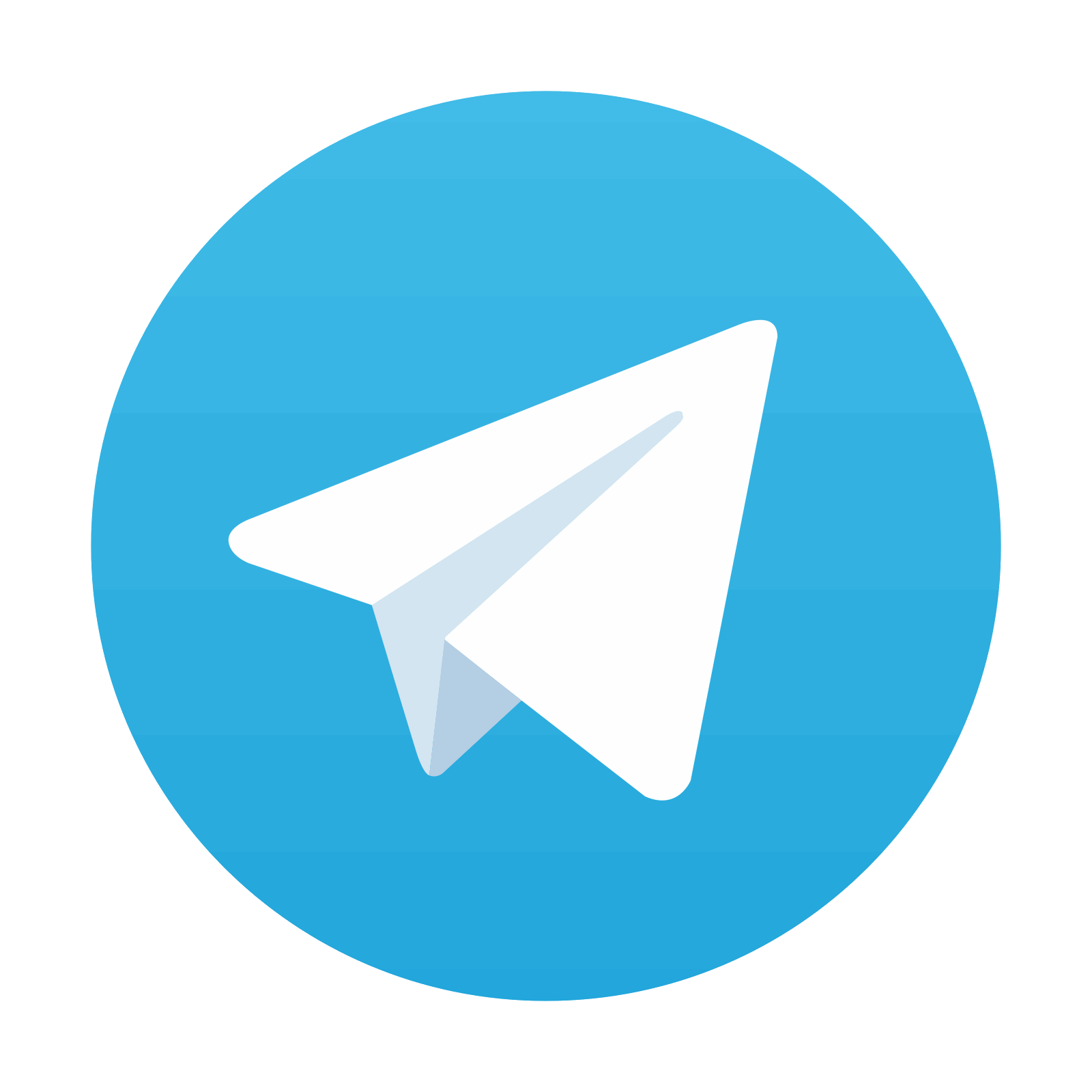
Stay updated, free articles. Join our Telegram channel

Full access? Get Clinical Tree
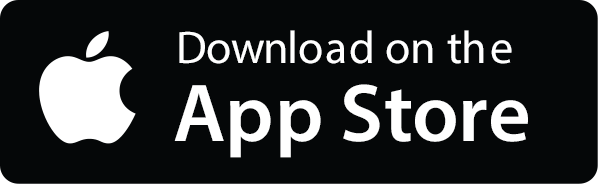
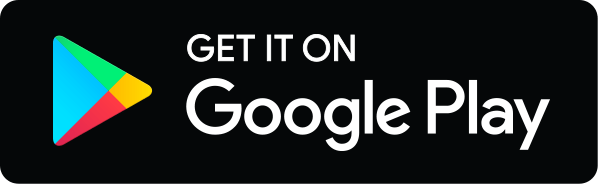