21 Colon Carcinoma: Epidemiology, Etiology, Pathology, and Diagnosis
Abstract
Colorectal carcinoma is the third most common internal malignancy, and the second leading cause of carcinoma death.
The incidence and mortality has declined in recent years, most likely due to increased screening. This chapter reviews the incidence, prevalence, epidemiology, etiology, pathology, and diagnosis of malignant colonic neoplasia.
21.1 Classification
Malignancies of the large intestine assume a major importance because of their frequency in the general population. The types of malignancies and their estimated incidence are listed in ▶ Table 21.1. 1 , 2
Histology | DiSario et al 1 | (%) Kang et al 2 (%) |
Adenocarcinoma | 94.5 | 97 |
Carcinoid | 3.3 | 1.5 |
Lymphoma | 0.3 | 0.6 |
Sarcoma | 0.1 | |
Squamous cell carcinoma | 0.1 | 0.3 |
Plasmacytoma | ||
Melanoma | 0.03 |
21.2 Adenocarcinoma
21.2.1 Incidence, Prevalence, and Trends
Colorectal carcinoma is the third most common internal malignancy, and the second leading cause of carcinoma death. 3 It was estimated that in 2017, there would be 95,520 new cases of colon and 39,910 cases of rectal carcinoma in the United States and that 50,260 deaths would result from this disease. 3 The incidence and mortality has declined in recent years most likely due to increased screening. The overall lifetime risk of developing colorectal cancer is 4.7% in men and 4.4% in women.
In the United States, over the past several decades the survival rate has improved for each stage. The current 5-year relative survival for people with stage I colon cancer is about 92%, stage IIA 87%, stage IIB 63%, stage IIIA 89%, stage IIIB 72%, stage IIIC 53%, and stage IV 11%. 3
21.2.2 Epidemiology
An extensive and comprehensive review of the worldwide information on colon carcinoma was collated by Correa and Haenszel. 4 Much of the following information was extracted from their excellent review.
Age
Carcinoma of the large intestine is predominantly a disease of older patients, with the peak incidence being in the seventh decade. However, it must be borne in mind that the disease can occur at virtually any age and may be seen in patients in their 20 s and 30 s. 5 It has been estimated that only 5% of colorectal carcinomas occur in patients who are younger than 40 years of age. 6
Family History
There have been many reports that indicate an increased incidence of colorectal carcinoma in first-order relatives of patients who have suffered from the disease. In a prospective study of 32,085 men and 87,031 women, Fuchs et al 7 found that the age-adjusted relative risk (RR) of colorectal carcinoma for men and women with affected first-degree relatives, when compared with those without a family history of the disease, was 1.72. The RR among study participants with two or more first-degree relatives was 2.75. For participants younger than 45 years, who had one or more affected first-degree relatives, the RR was 5.37.
To compare the risk in relatives of patients with colorectal carcinoma diagnosed at different ages, Hall et al 8 studied two cohorts of patients, 65 diagnosed when they were younger than 45 years of age and 212 patients of all ages. The overall RR of colorectal carcinoma in first-degree relatives was 5.2 in the first group and 2.3 in the second group. The cumulative incidence of colorectal carcinoma for relatives of the young cohort rose steeply from 40 years, reaching 5% at 50 years and 10% at 70 years, compared with the older group, reaching 5% at 70 years and 10% at 80 years.
St John et al 9 conducted a case-control family study of 7,493 first-degree relatives and 1,015 spouses of 523 case-control pairs to determine the RR of developing carcinoma. The authors found an odds ratio (OR) of 1.8 for one and 5.7 for two affected relatives. The risk to parents and siblings was 2.1 times greater, 3.7 for patients diagnosed before 45 years and 1.8 times greater for patients diagnosed at the age of 45 years or older. The cumulative incidence was 11.1, 7.3, and 4.4% among relatives 55 years and older, between 45 and 54 years, and younger than 45 years, respectively. The most recent summary has shown the risk to be increased twofold to fourfold (▶ Table 21.2). 10 , 11 , 12 , 13 Furthermore, people who have a first-degree relative with colorectal carcinoma are estimated to have an average onset of colorectal carcinoma about 10 years earlier than people with sporadic colorectal carcinoma. 9
Site
The distribution of carcinoma in the various segments of the large bowel has been the subject of several detailed clinical studies. Each of these studies has shown that over the past 50 years there has been a gradual shift in the location of carcinomas from the rectum and left colon toward the right colon. Studies by Mamazza et al 11 and Obrand et al 12 further documented that the left-to-right progression has continued. Reasons for this shift to the right are not entirely clear. A review of patterns in different countries has revealed an increase in the incidence of colon carcinoma with a corresponding decrease in rectal carcinoma. 4 Such findings imply that methods for the early detection and screening of large bowel carcinoma should be directed at the entire colon rather than being limited to the distal 25 cm of the large intestine. Qing et al 13 in a comparison between American and Chinese patients found lesions in 36.3% of white patients versus 26.0% of Asian patients, while carcinomas of the rectum were found in 63.7% of white patients and 74% of Asian patients. The rightward shift has continued in recent decades in the United States 14 and Japan. 15
Geographic Distribution
There is a wide variation of the incidence of colorectal carcinoma in different countries. In general, countries of the Western world have the highest incidence of colorectal carcinoma, and these include Scotland, Luxembourg, Czechoslovakia, New Zealand, Denmark, and Hungary.
Countries with the lowest incidence include India, El Salvador, Kuwait, Martinique, Poland, and Mexico. The United States and Canada hold an intermediate position. 3 , 4 In large countries extending over a wide range of latitudes, there may be considerable regional differences that mimic international variations. 4
It has been suggested that low-risk populations have a relatively increased incidence of right-sided carcinomas, while relatively high-risk communities have an increased risk of left-sided malignancies. 4 There is an increased risk of large bowel carcinoma in urban populations when compared to rural populations. The incidence of colorectal carcinoma in Japanese Americans is higher than in Japanese individuals living in Japan. The children of these immigrants have an incidence approximating that of the general U.S. population. The effects of environmental exposure and food habits can be exemplified by a notable occurrence in Israel. Israelis who were born in Europe or North America run roughly 2.5 times the risk of bowel carcinoma of those born in North Africa or Asia. After their arrival in Israel, the incidence becomes similar. 4
Race and Religion
Black Americans who once enjoyed a lower incidence of colorectal carcinoma than their white counterparts now suffer a similar incidence of the disease, 4 but the 5-year survival rate for African-Americans is significantly lower than for whites. 16 The risk of large bowel malignancy in American Indians is less than half that for whites in the United States. Individuals of Mexican extraction born in the United States also experience a lower risk for large bowel carcinoma. With respect to religion, Jews in the United States have a higher incidence of colorectal carcinoma, while Mormons and Seventh Day Adventists have a lower rate than the general U.S. population. 4 Ashkenazi Jews have a lifetime colorectal carcinoma risk of 9 to 15%, which differs strikingly from the 5 to 6% colorectal carcinoma risk for non-Ashkenazi members of the general western populations. 17 The lower incidence in Mormons has been attributed to their prohibition of the use of tobacco and alcohol. Self-reported or perceived religiousness has been determined to be a protective factor in the development of colorectal carcinoma (an RR of 0.7). 18
Occupation
Vobecky et al 19 observed an increased RR of three (i.e., a threefold increase) in the incidence of colorectal carcinoma in individuals working in factories that produce synthetic fibers. In the authors’ review of the literature, they found that other workers who were at greater risk of developing large bowel carcinoma included metallurgy workers handling chlorinated oil, manufacturers of transport equipment, weavers, firemen, those working with asbestos or coke by-products, and those working in copper smelters. de Verdier et al 20 found elevated RR of colon carcinoma among male petrol station and/or automobile repair workers (2.3) and men exposed to asbestos (1.8), while elevated RR of rectal carcinoma was found among men exposed to soot (2.2), asbestos (2.2), cutting fluids and/or oils (2.1), and combustion gases from coke, coal, and/or wood (1.9).
A meta-analysis by Homa et al 21 suggested that exposure to amphibole asbestos may be associated with colorectal carcinoma, but exposure to serpentine asbestos is not. Another study failed to find an association between asbestos exposure and carcinoma of the colon and rectum. 22 Cumulative exposure to organic solvents, dyes, or abrasives also may contribute to an increased risk of colorectal carcinoma. 23 , 24 Workers involved in the manufacture of polypropylene also exhibit an increased incidence of colorectal carnicoma, 25 but this risk was more recently reported not to exist. 26 Workers with intense exposure to ethyl acrylate and methyl methacrylate for 3 years have an increased risk of colon carcinoma two decades later. 27
21.2.3 Etiology and Pathogenesis
As with other malignancies, neither the etiology nor the pathogenesis of carcinoma of the colon is known. A number of factors have been considered important in its causation, and certain clinical conditions are considered precursors of carcinoma and will be detailed here.
Polyp–Cancer Sequence
Considerable evidence has accumulated to suggest that most, if not all, carcinomas develop from a precursor polyp, a situation known as the polyp–cancer sequence. This sequence is described in detail in Chapter 19.
Inflammatory Bowel Disease
Although colorectal carcinoma, complicating ulcerative colitis and Crohn’s disease, only accounts for 1 to 2% of all cases of colorectal carcinoma in the general population, it is considered a serious complication of the disease and accounts for approximately 15% of all deaths in inflammatory bowel disease patients.
Patients with universal ulcerative colitis, having a more severe inflammation burden and risk of the dysplasia-carcinoma cascade especially those who have had the condition for more than 10 years and those patients who experienced onset in childhood, without doubt are at increased risk of developing carcinoma of the colon or rectum. Lennard-Jones et al 28 reported that the incidence of colorectal carcinoma (in 22 among 401 patients with extensive ulcerative colitis followed over 22 years) was 3, 5, and 13% at 15, 20, and 25 years, respectively. For the 17 patients developing colorectal carcinoma during supervised surveillance (344 patients), Dukes’ staging was A or B in 12 patients. In half the carcinoma patients under surveillance, dysplasia signaled the associated carcinoma found only after colectomy in the operative specimens. Others have confirmed the increased risk. 29 Of 3,117 patients with ulcerative colitis followed for up to 60 years through the Swedish Cancer Registry, the RR of colorectal carcinoma was 5.7 (nonsignificant for proctitis), 2.8 for left-sided disease, and 14.8 for pancolitis. 30 Recent figures suggest that the risk of colon carcinoma for people with inflammatory bowel disease increases by 0.5 to 1.0% yearly, 8 to 10 years after diagnosis. 31 Considering the chronic nature of the disease, it is remarkable that there is such a low incidence of colorectal carcinoma in some of the population-based studies, and possible explanations have to be investigated. One possible carcinoma protective factor could be treatment with 5-aminosalicylic acid preparations (5-ASAs). 31
Adenocarcinoma of the small bowel is extremely rare, compared with adenocarcinoma of the large bowel. Although only few small bowel carcinomas have been reported at sites of involvement with Crohn’s disease, the number was significantly increased in relation to the expected number. 31 The incidence of colorectal carcinoma in patients with Crohn’s disease has been reported as being 4 to 20 times greater than the general population. 29 In a study of 1,656 patients with Crohn’s disease, Ekbom et al 32 indicated RR for colon carcinoma of 3.2 (in Crohn’s ileocolitis) to 5.6 (in Crohn’s colitis only). With the onset of any Crohn’s colitis before the patient was 30 years of age, the RR was 20.9, but only 2.2 when diagnosed after age 30 years.
Genetics
In the last decade and a half, there has been an explosive increase in knowledge about the molecular biology of carcinoma. Publications are legion but often difficult to understand. This notwith-standing, the past decade has been witness to unprecedented progress in the comprehension of the basic mechanisms involved in the genesis of colorectal carcinoma. In his outstanding review of the molecular biology of colorectal carcinoma, Allen 33 attempted to make the subject understandable to the clinician, and the following dissertation draws heavily from that review.
Molecular Biology
The codes that control production of protein enzymes and form the basic information needed for life itself are found within the cell nucleus as long strands of deoxyribonucleic acid (DNA) molecules that are, in turn, composed of four nucleotides: adenine (A), guanine (G), thymine (T), and cytosine (C). Under normal circumstances, adenine only pairs with thymine (A:T), and guanine pairs with cytosine (G:C). As a result of base pairing, cellular DNA forms the familiar stepladder configuration that is twisted into a double helix and supercoiled into microscopically visible structures called chromosomes.
Long DNA sequences are subdivided into smaller segments called genes, each of which contains the information needed for a single protein. Genes are composed of hundreds or thousands of nucleotides. In humans, the entire genetic code, termed the genome, is composed of approximately 3 billion nucleotides organized into approximately 100,000 genes contained within 23 pairs of chromosomes (total, 46). One chromosome in each pair is inherited from the mother and one from the father. Thus, each gene has another similar (but not identical) gene called an allele on the complementary chromosome. Genes can act in a dominant or recessive fashion. For dominant genes, one allele assumes the responsibility for producing the protein and the other allele remains dormant.
The sequence of nucleotides within cellular chromosomes is reproduced faithfully and is passed down from generation to generation during cell division. A “normal” rate of mutation is estimated to be one mistake in every 10 billion base pairs copied. To correct replication errors (RER), a repair mechanism is dependent on genes called mismatch repair genes. Malignant transformation appears to result from the accumulation of mutations within genes that are critical to cell growth and differentiation caused either by an increase in the mutational rate or because the DNA repair process is compromised. A carcinoma is the end result of 4 to 12 genetic changes that convey a growth advantage to the mutated cells. During the initiation phase, there is an increase in the mutational rate of DNA. Mutations in some genes become incorporated into an individual’s genome and are passed from generation to generation. These “germline mutations” may occur in genes related to carcinoma and, as a result, cause hereditary carcinoma. Other mutations, termed “somatic,” cause a sporadic carcinoma. Knudson 34 proposed that inherited carcinomas arise in individuals with germline mutations of one allele of a recessively acting carcinoma gene, after which only one additional somatic alteration is needed to inactivate the gene and initiate carcinogenesis. Sporadic carcinomas require two somatic mutations (or allelic loss).
Mechanisms of Gene Action
Three major categories of genes have been implicated in carcinoma development: oncogenes, 1 tumor suppressor genes, 2 and mismatch repair genes 3 (▶ Table 21.3). When a proto-oncogene (a normal human growth–related gene) becomes abnormally activated, it drives the cell through the cell cycle facilitating clonal proliferation and is known as an oncogene. Oncogenes act in a dominant fashion because alteration of only one allele is necessary to produce a cellular effect. Oncogenes, however, do not tell the entire story, because only 20% of human carcinomas carry oncogene alterations.
Other genes called tumor suppressor genes can halt the cell cycle even when oncogenes are altered. Tumor suppressor genes act in a recessive manner and promote carcinoma only when they are inactivated by allelic loss or mutations in both alleles. If cells cannot repair DNA damage, tumor suppressor genes such as p53 drive the cell into a suicide mode called apoptosis. A tumor suppressor gene critical to colorectal carcinoma was described on chromosome 5—the adenomatous polyposis coli (APC) gene. It was found to contain an inherited mutation causing truncation of the protein product. Somatic mutations of APC are found early during the neoplastic process in most polyps and carcinomas.
The latest genes found to be related to carcinogenesis are called mismatch repair genes, which are needed for cells to repair DNA RER and spontaneous base pair loss. The six DNA mismatch repair genes found in humans to date are hMSH2 (chromosome 2p16), hMLH1 (chromosome 3p21), hPMS1 (chromosome 2q31–33), hPMS2 (chromosome 7q11), hMSH6 (chromosome 2p16), and hMSH3 (chromosome 5q11.2-q13.2). When both copies of these genes are inactivated, DNA mismatch repair is defective, and the cell exhibits an increased frequency of mistakes in DNA replication, thereby accelerating the progression to oncogenesis. The first four genes are regarded to contribute to hereditary nonpolyposis colorectal carcinoma (HNPCC) in 31, 33, 2, and 4%, respectively. 35
The founder mutation MSH2*1906G > C is also considered an important cause of HNPCC in the Ashkenazi Jewish population. 36 This pathogenic mutation accounting for 2 to 3% of colorectal carcinoma in those whose age at diagnosis is less than 60 years is highly penetrant and accounts for approximately one-third of HNPCC in Ashkenazi Jewish families that fulfill the Amsterdam criteria. This founder mutation MSH2*1906 was found in 8% of 1,342 individuals (0.6%) of those of Ashkenazi descent with colorectal carcinoma. A subsequent study 37 sought to characterize the proportion of individuals of Ashkenazi heritage with very early-onset colon carcinoma (diagnosed at age 40 years or younger) that could be attributed to MSH2*1906G > C detected the mutation in 3 of the 41 samples (7.14%) of patients who had colorectal carcinoma diagnosed at age 40 years or younger. The incidence is significantly greater than the 8 in 1,345 (0.6%) observed for cases of colorectal carcinoma in Ashkenazi Jews not selected for age. These results suggest that consideration for testing for the MSH2*1906G > C mutation should be included in the evaluation of Ashkenazi Jewish individuals diagnosed with early onset of colon carcinoma. 38
hMSH2 and hMLH1 accounted for 63% of kindreds meeting international diagnostic criteria. 38 A recent review by Peltomäki 39 cited germline mutations in one of four major HNPCC-associated mismatch repair genes (MLH1, MSH2, MSH6, and PMS2) detected in up to 70 to 80% of such families. More than 400 different predisposing mismatch repair gene mutations are known with approximately 50% effecting MLH1, about 40% MSH2, and about 10% MSH6. 39 The share of PMS2 is less than 5%. The newly identified human mismatch repair gene MLH3 may account for a small percentage of HNPCC. A germline mutation in PMS1 was originally reported in an HNPCC-like family, but there is presently no evidence of PMS1 as an HNPCC predisposition gene. The available data on two additional components of mismatch repair, exonuclease 1 (EXO1) and DNA polymerase, are too limited to allow any reliable assessment of their role in HNPCC predisposition. 39
Genetic Pathways to Colorectal Carcinoma
Traditionally, carcinoma is seen as a three-step process of initiation, promotion, and progression. Colorectal carcinoma is a genetically heterogeneous disease, and a series of genetic events has been described in the evolution of colorectal carcinoma. The initiation stage (from the beginning to the first mutation) involves a complex (and poorly understood) interplay between environmental factors and host susceptibility (▶ Fig. 21.1). Specific environmental factors are known to modify colorectal carcinoma (▶ Fig. 21.2). For patients with hereditary colorectal carcinoma, the influence of environmental factors is small compared with the power of the underlying genetic mutations. Thus, the risk of initiating colorectal carcinoma is substantially higher in hereditary conditions (100% in patients with polyposis syndromes and approximately 85% in HNPCC).


A number of early reports tried to relate genetic importance to the etiology of colorectal carcinoma. Burt et al 40 examined the inheritance of susceptibility to colonic polyps and carcinomas in a large pedigree with multiple cases of colorectal carcinoma. The authors’ analysis suggested that the observed excess of discrete adenomatous polyps and colorectal carcinoma was the result of an inherited autosomal-dominant gene for susceptibility rather than an inherited recessive gene for susceptibility. Solomon et al 41 examined colorectal carcinomas for loss of alleles on chromosome 5. Using a special probe that maps to chromosome 5q, the authors demonstrated that at least 20% of carcinomas lose one of the alleles present in matched normal tissue. They suggested that becoming recessive for this gene may be a critical step in the progression of a relatively high proportion of colorectal carcinomas. No deletions were found in any other chromosome, which indicates that the loss from chromosome 5 is nonrandom. Law et al 42 reported allelic losses in chromosomes 17 and 18 to be more frequent in colorectal carcinoma than losses on chromosome 5.
It is now believed that the mutation that initiates colonic neoplasia is found in one of two gene loci. The 5q21 loci contain the APC gene, which is altered in more than 70% of all neoplastic lesions. Other polyps and carcinomas demonstrate microsatellite instability (MSI), a hallmark of mismatch repair gene mutations. Depending upon which type of gene has been inactivated, one of two pathways to colorectal carcinoma is followed (▶ Fig. 21.1). In the first pathway, APC gene inactivation leads to a pathway termed loss of heterozygosity (LOH). Approximately 70 to 80% of colorectal carcinoma develops through the LOH pathway following inactivation of the APC gene. The genes involved in the LOH pathway include K-ras, deleted in colon carcinoma (DCC), and p53 in addition to APC (▶ Fig. 21.3). Germline APC mutations initiate the neoplastic process in patients with familial adenomatous polyposis (FAP) and endow all colonic crypt stem cells with a high risk for clonal proliferation.

A large body of evidence supports the concept of a multistep process that typically develops over decades and appears to require at least seven genetic events for completion. But even single altered genes can result in disease (e.g., FAP, HNPCC). In 1990 Fearon and Vogelstein 43 published the now-classic genetic model for colorectal carcinogenesis. The authors proposed a genetic series of events that corresponded to the apparent ordered sequence from a benign to a malignant lesion in histopathologic recognizable stages. They postulated that colorectal carcinoma arises as a result of mutational activation of oncogenes coupled with the mutational inactivation of tumor suppressor genes. Their original suggestion was that there must be mutations in at least four or five genes, but it is now believed to be at least seven for the formation of a carcinoma. Although the genetic alterations often occur according to a preferred sequence, the orderly sequence (▶ Fig. 21.2 and ▶ Fig. 21.3) rarely occurs in any individual carcinoma. It is the total accumulation of genetic alterations rather than their order that is responsible for determining the biologic properties of the carcinoma. The cascade of events described by Fearon and Vogelstein 43 begins with a loss or mutation of the FAP gene on chromosome 5q, resulting in a change from normal epithelium to hyperproliferative epithelium. One of these hyperproliferating cells gives rise to a small adenoma in which the genome is hypomethylated. The next event involves activation of the K-ras oncogene on the chromosome 12p mutation to form the intermediate adenoma. Unlike oncogenes, tumor suppressor genes are expressed in a recessive manner. Therefore, both allelic copies must be lost or inactivated by point mutations for phenotypic expression to occur. Usually, the DCC gene on chromosome 18q is next to be deactivated or lost, and results in the development of a late adenoma. The final genetic alteration found consistently in colorectal carcinoma is loss and/or mutation of the p53 tumor suppressor gene on chromosome 17p. The p53 gene is altered in 50% of all human carcinomas and in 70% of colorectal carcinomas. Further genetic alterations are required for the development of metastases, subsequently believed to involve the LOH of the Nm23 gene. 44 While there is no obligatory sequence of mutations in the pathway from normal mucosa through adenoma to carcinoma, there is clearly an association of certain types of mutations in specific oncogenes or tumor suppressor genes with early and late states of transformation. This multistep pathway can be observed in sporadic and inherited colorectal carcinoma. Many other genes, such as MCC, TGF-β, Rb, and Myc, have been implicated in the genesis of colorectal carcinoma. Further study of the molecular events will undoubtedly lead to a better understanding of the multistep carcinogenesis and the relative importance of each.
Mismatch repair gene defects initiate an entirely different sequence of events known as the RER pathway. These pathways lead to carcinomas that are biologically quite different. This second pathway to colorectal carcinoma is found in approximately 20% of carcinomas. The RER pathway is similar in both patients with HNPCC and those who develop a spontaneous RER carcinoma. Patients with HNPCC inherit a single defective allele of a mismatch repair gene and require an additional somatic mutation to inactivate the second allele. Spontaneous carcinomas develop after two somatic events inactivate the relevant gene. In either case, inactivation leads to a marked increase in RER. As errors accumulate in microsatellites, malfunction of genes that contain or are near affected microsatellites may occur (▶ Fig. 21.4). Aaltonen et al 45 found the RER-positive phenotype in 77% of colorectal carcinomas from HNPCC patients compared with only 13% of patients with sporadic carcinoma.

How do DNA mismatch repair defects cause carcinoma? The mismatch repair gene defect increases the risk of malignant transformation of the cells, which may ultimately result from the disruption of one or several anticarcinogenic functions of the mismatch repair genes. Peltomäki recently summarized these. 39 First, malfunction of the mismatch repair system is associated with decreased genomic stability, which may manifest itself as highly elevated rates of subtle mutations (MSI) throughout the genome. Second, although mismatch repair–deficient cells typically have a diploid or near-diploid DNA content, loss of heterology-dependent suppression of recombination in these cells may promote gene conversion and expose tumor suppressor genes in analogy to LOH, or allow chromosomal translocations to occur. Furthermore, increased mutational inactivation of genes involved in DNA double-strand break repair may contribute to an elevated degree of chromosomal aberrations in mismatch repair–deficient cells. Third, besides anonymous microsatellite sequences, critical genes may be affected with mutations, conferring a growth advantage on the cells. Typical “target” genes include those involved in growth suppression, apoptosis, or signal transduction. Fourth, there is evidence that failed protection against endogenous or exogenous DNA damage and the ensuing persistence of mutagenic or premutagenic lesions may contribute to genomic instability/MSI in intestinal cells.
There are examples of non-FAP, non-HNPCC hereditary colorectal carcinomas; a family not meeting the Amsterdam criteria with no deficiency of DNA mismatch repair in the malignancy of the proband was found to have a germline mutation of the TGFBR2 gene that encodes the type II TGF-β receptor; loss of the remaining normal allele was observed in the carcinoma. 46 Thus, other biologic mechanisms may underlie non-FAP, non-HNPCC hereditary colorectal carcinoma.
The transition from normal epithelium to adenoma to carcinoma is associated with acquired molecular events. A recent update on the genetic pathway to carcinoma has become available. 13 At least five to seven major molecular alterations may occur when a normal epithelial cell progresses in a colonal fashion to carcinoma. There are at least two major pathways by which these molecular events can lead to colorectal carcinoma. About 85% of colorectal carcinomas are due to events that result in chromosomal instability and the remaining 15% are due to events that result in MSI. Key changes in chromosomal instability carcinomas include widespread alterations in chromosome number (aneuploidy) and detectable losses at the molecular level of portions of chromosome 5q, chromosome 18q, and chromosome 17p; and mutation of the KRAS oncogene. The important genes involved in these chromosome losses are APC (5q), DCC/MADH2/MADH4 (18q), and TP53 (17p), respectively, and chromosome losses are associated with instability at the molecular and chromosomal level. Among the earliest events in the colorectal carcinoma progression pathway is loss of the APC gene, which appears to be consistent with its important role in predisposing persons with germline mutations to colorectal neoplasms. Acquired or inherited mutations of DNA damage repair genes also play a role in predisposing colorectal epithelial cells to mutations. Not every carcinoma acquires every mutation, nor do mutations always occur in a specific order. The key characteristics of MSI carcinomas are largely intact chromosome complement, but acquisition of defects in DNA repair, such that mutations that may occur in important carcinoma-associated genes are allowed to persist. These types of carcinomas are detectable at the molecular level by alterations in repeating units of DNA that occur normally throughout the genome, known as a DNA microsatellite. Mitotic instability of microsatellites is the hallmark of MSI carcinomas.
MSI, the hallmark of HNPCC, occurs in approximately 15 to 25% of sporadic colorectal carcinomas. According to international criteria, a high degree of MSI (MSI-H) is defined as instability at two or more of five loci or ≥ 30 to 40% of all microsatellite loci studied, whereas instability at fewer loci is referred to as MSI-low (MSI-L). Colorectal carcinomas with MSI-H encompass a group of malignancies with a predilection for the proximal colon, that have diploid DNA content, that are high grade, that are associated with female sex, and have better survival. These features distinguish MSI-H carcinomas from those without widespread MSI, that is, MSI-L or microsatellite-stable (MSS) carcinomas. A majority of MSI-H colon carcinomas are caused by inactivation of MLH1. Whereas the MSI-L subset of colon carcinomas is equally as prevalent as the MSI-H group, immunohistochemical and mutation studies have found no involvement of MLH1, MSH2, MSH6, or MSH3 in the former carcinomas. The clinicopathological features do not seem to distinguish this group from MSS colon carcinomas either.
Clinical Relevance of Basic Genetic Knowledge
An effort by Allen 33 to reclassify the subtypes of colorectal carcinoma based on their molecular pathogenesis and inheritance pattern is depicted in ▶ Table 21.4. Prior to the definition of molecular pathways to colorectal carcinoma, proximal carcinomas were known to have normal cytogenetics, diploid DNA content, slower growth, less frequent metastasis, and a better prognosis compared to distal carcinomas. In addition, the frequency of extracolonic carcinomas had been found to be higher in both patients with colorectal carcinoma and their first-degree relatives when the index colon carcinoma was proximal.
Most carcinomas that arise in the distal colon develop along the LOH pathway, while most proximal carcinomas are RER (▶ Fig. 21.5). Clinical characteristics of LOH carcinomas induce a propensity for left-sided location (80%), aneuploidy, a polyp-tocarcinoma ratio of 20:1, and a total developmental period of 7 to 10 years. The exception to this observation is the rare adenomatous polyposis syndrome variant called hereditary flat adenoma syndrome (HFAS) or attenuated adenomatous polyposis coli (AAPC) syndrome associated with APC mutations. In this syndrome, the inherited point mutation is found upstream from FAP mutations within exons 1 to 4. This subtle difference between FAP and AAPC mutations (sometimes within 10 base pairs of each other) results in dramatically different phenotypes. Patients with AAPC have few polyps (usually fewer than 10); the polyps are diminutive, flat, and located in the proximal colon; and a high proportion of polyps progress to carcinomas and they develop late compared with other hereditary neoplasias related to APC mutations. RER carcinomas tend to develop proximal to the splenic flexure (> 70%), have a normal (diploid) DNA content, and carry a better prognosis compared to LOH carcinomas. Molecular analysis of resected carcinomas has led to recognition that LOH carcinomas carry a worse prognosis, stage for stage, than RER carcinomas. To date, the most important molecular marker for prognosis appears to be the DCC gene locus on chromosome 18.

Classification based on genetic patterns and specific syndromes reveals three major forms: sporadic, familial, or inherited. The difference appears to be the method by which the initiating mutation occurs. For sporadic carcinomas, there is a period during which environmental factors influence colon mucosa and eventually alter it so that clonal growth can occur. Familial colorectal carcinoma is defined by patients who have several family members with colon or rectal carcinoma but who do not fit a recognized inherited pattern. Familial colorectal carcinoma may include as many as 30% of patients with colorectal carcinoma when both carcinoma and polyps are included in the pedigree. Explanations for familial clustering could be either shared environment, shared genetics, or both.
Liu et al 47 divided carcinomas with MSI into three classes. The first subset of colorectal carcinomas occurs in patients without a strong family history of colorectal carcinoma (sporadic cases). These account for 12 to 15% of the total colorectal carcinomas in the United States. The second class comprises colon, endometrial, and ovarian carcinomas that develop in patients with a family history of these forms of carcinoma (HNPCC). Virtually all carcinomas that develop in these patients exhibit MSI. The third class of RER carcinomas includes a variable fraction of several types of neoplasms, including those of the lung, breast, and pancreas. The magnitude and prevalence of the microsatellite alterations in this class are generally less pronounced than in RER colorectal carcinomas. The results of Liu et al led to three major conclusions. First, carcinogenesis associated with MMR gene defects usually results from the inactivation of both alleles of the relevant gene. Second, a significant fraction of sporadic RER carcinomas (four of seven in study) arises from mutations in genes other than the four that account for most HNPCC cases. Finally, most sporadic RER carcinomas are not associated with germline mutations of the known match repair genes.
In their review, DeFrancisco and Grady 48 cited the causative genes of HNPCC in decreasing frequency of occurrence to include MLH1, MSH2, MSH6, MLH3, PMS1, PMS2, TGFBR2, and EXO1. Peltomäki summarized the phenotypic features associated with germline mutations manifesting a predisposition to HNPCC. 39 MLH1 is mostly associated with typical HNPCC. Approximately 30% of mutations are of the missense type whose phenotypic manifestations may vary. MSH2 is also mostly associated with typical HNPCC. Extracolonic carcinomas may be more common than in MLH1 mutation carriers. It is a major gene underlying the Muir–Torre syndrome. MSH6 is associated with typical or atypical HNPCC. It is often characterized by late onset, frequent occurrence of endometrial carcinoma, distal location of colorectal carcinomas, and low degree of MSI in carcinomas. PMS2 is also associated with typical or atypical HNPCC. The penetrance of mutations may vary. It is a major gene underlying Turcot’s syndrome. MLH3 is seen mostly in atypical HNPCC. It may be characterized by distal location of colorectal carcinomas and variable degrees of MSI in carcinomas. EXO1 is mostly seen in atypical HNPCC. It may be associated with MSI in carcinomas.
Lawes et al 49 reviewed the world literature to determine the clinical importance and prognostic implications of MSI and sporadic carcinoma. In clinical studies, colorectal carcinomas demonstrating MSI respond better to chemotherapy, while in vitro studies using MSI positive cell lines show resistance to radiotherapy and chemotherapy. They concluded MSI may be a useful genetic marker in prognosis and could be an influential factor in deciding treatment options.
Clinical Syndromes
For many years, FAP was considered the only hereditary variety of colon carcinoma. Now, three types of hereditary colorectal carcinomas are recognized: adenomatous polyposis syndromes (Chapter 19), HNPCC, and familial colorectal carcinoma, a group in which families exhibit aggregation of colorectal carcinoma, and/or adenomas, but with no identifiable hereditary syndrome. Approximately 1 to 3% of all colorectal carcinomas are due to hereditary adenomatous polyposis syndromes that include FAP, Gardner’s syndrome, Turcot’s syndrome, and HFAS/AAPC, all of which begin with germline mutations in the APC gene.
Hereditary Nonpolyposis Colorectal Carcinoma
HNPCC is inherited as an autosomal-dominant pattern with almost complete penetrance. It is estimated that from 0.5 to 6.0% of all colorectal carcinomas can be attributed to HNPCC. 35 , 50 , 51 , 52
Based on clinical criteria only, Peltomäki 36 noted the estimated incidence of HNPCC varies between 0.5 and 13% of the total colorectal carcinoma burden. Strictly molecular approaches based on the identification of germline mutation carriers among newly diagnosed colorectal patients whose carcinomas showed MSI have arrived at lower estimates—0.3 to 3% of the total colorectal carcinoma burden.
HNPCC has four major subtypes: (1) Lynch type I (site-specific nonpolyposis colorectal carcinoma); (2) Lynch type II (formerly called the carcinoma family syndrome), in which carcinomas occur in the colon and related organs (endometrium, ovaries, stomach, pancreas, and proximal urinary tract, among others); (3) Muir–Torre syndrome, associated with multiple benign and malignant skin neoplasms, sebaceous gland adenomas, carcinomas, and keratoacanthomas 53 ; and (4) a variant of Turcot’s syndrome (brain neoplasms). HNPCC-related colon carcinomas begin with mutations in the mismatch repair genes and are RER positive.
Clinical criteria were established to confirm the diagnosis of HNPCC at a meeting of the International Collaborative Group on HNPCC in Amsterdam in 1990. 51 The group agreed that minimum criteria should include (1) at least three relatives with histologically verified colorectal carcinoma, one of whom should be a first-degree relative of the other two (those with FAP should be excluded); (2) at least two successive generations should be affected; and (3) in one of the relatives, colorectal carcinoma should have been diagnosed when the patient was younger than 50 years of age.
These criteria have not proved to be comprehensive; therefore, in 1999, the International Collaborative Group developed revised criteria and these are known as Amsterdam-II criteria. They are essentially the same, except that there should be at least three relatives with HNPCC-associated carcinoma (colorectal, endometrial, small bowel, ureter, or renal pelvis). Even this extension of clinical features fails to identify some families with germline mismatch repair gene mutations, so the Bethesda guidelines for testing of colorectal carcinomas for MSI have been developed. They include the following:
Individuals with carcinoma in families that meet the Amsterdam criteria.
Individuals with two HNPCC-related carcinomas, including synchronous and metachronous colorectal carcinomas or associated extracolonic malignancies (endometrial, ovarian, gastric, hepatobiliary or small bowel carcinoma, or transitional cell carcinoma of the renal pelvis or ureter).
Individuals with colorectal carcinoma and a first-degree relative with colorectal carcinoma and/or HNPCC-related extracolonic malignancy and/or a colorectal adenoma; one of the malignancies diagnosed at age younger than 45 years, and the adenoma diagnosed at age younger than 40 years.
Individuals with colorectal or endometrial carcinoma diagnosed at age younger than 45 years.
Individuals with right-sided colorectal carcinoma with an undifferentiated pattern (solid/cribriform) on histopathology diagnosed at age younger than 45 years. (Note: solid/cribriform defined as poorly differentiated or undifferentiated carcinoma composed of irregular, solid sheets of large eosinophilic cells, and containing small glandlike spaces.)
Individuals with signet-ring-cell-type colorectal carcinoma diagnosed at age younger than 45 years. (Note: composed of 50% signet-ring cells.)
Individuals with adenomas diagnosed at age younger than 40 years.
In 2002, another workshop was held at the NCI (National Cancer Institute) in Bethesda; the revised guidelines that were created are listed in ▶ Table 21.5. 54 It is estimated that 20 to 25% of population-based cases of colorectal carcinoma meet the Bethesda criteria. It is suggested that for all patients who meet these criteria, a search for MSI is indicated. To establish the most effective and efficient strategy for the detection of MSH2/MLH1 gene carriers in HNPCC, Pinol et al 55 conducted a prospective multicenter nationwide study (the EPICOLON study) in 20 hospitals in the general community in Spain of 1,222 patients with newly diagnosed colorectal carcinoma. MSI testing and MSH2/MLH1 immunostaining were performed in all patients regardless of age, personal or family history, and carcinoma characteristics. Patients whose carcinoma exhibited MSI and/or lack of protein expression underwent MSH2/MLH1 germline testing. The revised Bethesda guidelines were fulfilled by 23.5% of patients and 7.4% had a mismatch repair deficiency, with the carcinoma exhibiting either MSI or loss of protein expression. Germline testing identified mutations in 0.9% in either MSH2 or MLH1 genes. Strategies based on either MSI testing or immunostaining previous selection of patients according to the revised Bethesda guidelines were the most effective (sensitivity, 81.8 and 81.8%; specificity, 98.0 and 98.2%; and positive predictive value, 27.3 and 29.0%, respectively) to identify MSH2/MLH1 gene carriers. They concluded the revised Bethesda guidelines are the most discriminating set of clinical parameters (OR, 33.3).
In conclusion, MSI and immunohistochemistry analysis using antibodies against MLH1, MSH2, PMS2, and MSH6 appeared to be equally effective for the identification of mutation carriers. Despite its name, polyps are a feature of HNPCC, and a review of the literature revealed a polyp incidence in 8 to 17% of first-degree relatives during colonoscopic screening. 38
Carriers of an MMR defect develop adenomas more frequently than controls. The adenomas identified in carriers are larger, and a significantly higher proportion showed histologic features that are associated with a high risk of malignant degeneration, such as a high degree of dysplasia and the presence of more extensive villous architecture. 56 A relatively high proportion of patients develop colorectal carcinoma within 3 years after a clean colonoscopy and this suggests that the adenoma–carcinoma sequence is accelerated and that the progression from adenoma to carcinoma may take fewer than 3 years.
Genetically speaking, HNPCC syndromes are dominantly inherited with nearly 100% penetrance reported by one group of investigators 50 but only 70 to 80% (i.e., 20–30% of individuals with a predisposing mutation may never develop carcinoma) by others. 57 Asymptomatic gene carriers can pass the causative mutation to their children. 57 The disease is heterogeneous. All first-degree relatives of a patient with HNPCC have a 50% risk of carrying one of the deleterious genes. Adenomas in patients carrying HNPCC gene mutations display MSI, suggesting that mismatch repair defects are important early events in colorectal carcinogenesis. Carcinoma formation requires inactivation of both copies of a given mismatch repair gene, one copy by germline mutation and the other by somatic (acquired) mutation. 57
Individuals with the Lynch syndrome differ from patients with sporadic colorectal carcinoma in several ways. 35 They show an autosomal-dominant mode of inheritance, 1 a predominance of proximal colonic carcinoma (72% of first colon carcinomas were located in the right colon, and only 25% were found in the sigmoid colon and rectum), 2 an excess of multiple primary colonic carcinomas (18%), 3 an early age of onset (mean, 44 years), 4 a significantly improved survival rate compared with family members with distal colonic and rectal carcinomas when compared with right-sided lesions in an American College of Surgeons series (53% vs. 35% 5-year survivals), 5 and 24% developed metachronous colon carcinoma with a risk for the development of metachronous lesions in 10 years of 40% based on life-table methods. 6 In addition to the above features, Lynch type II syndrome is characterized by a high frequency of other adenocarcinomas and the occasional occurrence of cutaneous manifestations in the form of sebaceous adenomas and carcinomas, epitheliomas, or keratoacanthomas. In a study by Mecklin and Jarvinen, 58 of 40 HNPCC families with 315 affected members, a total of 472 malignancies were identified. They included colorectal (63%), endometrial (8%), gastric (6%), biliopancreatic (4%), and uroepithelial (2%) carcinomas.
The RR of these carcinomas ranges from 3 to 25 times that of the general population. 10 The risk of colorectal carcinoma increases 1.6% per year from age 25 to 75 years in patients with these mutations. 53 In a detailed pedigree analysis of 40 families with HNPCC, Aarnio et al 59 identified 414 patients affected with carcinoma. The risk of any metachronous carcinoma reached 90% after treatment of colorectal carcinoma and 70% after endometrial carcinoma; the second malignancy was most often a new colorectal carcinoma or endometrial carcinoma. 59 Other sites of carcinoma include breast, pancreas, and possible lymphoma and leukemia. The review by DeFrancisco and Grady 48 found the four most common extracolonic carcinomas include (in descending order) endometrial, ovarian, gastric, and transitional cell carcinoma of the uroepithelial tract (bladder, kidney, ureter). Women with HNPCC are at a 10-fold increased risk of endometrial carcinoma, which is usually diagnosed between the ages of 40 and 60 years, that is, 15 years earlier than the general population. The estimated cumulative risk at age 70 years is 40 to 50%. With MSH2 and MLH1 mutations, the risk of colorectal carcinoma or endometrial carcinoma before age 50 years is 20 to 25% compared with 0.2% for the general population. 53 Ovarian carcinoma is less common (incidence approximately 9%). Gastric carcinoma occurs in 5 to 20% of HNPCC families. The RR of gastric carcinoma was 19.3 in MSH2 mutation carriers compared with the general population. Transitional cell carcinoma of the uroepithelial tract occurs in 1% of HNPCC patients. Only the carriers of MSH2 mutations appear to have a significantly increased risk of carcinoma in the urinary tract (RR of 75.3). Overall, the RR of gastric carcinoma, ovarian carcinoma, and carcinoma of the urinary tract has been shown to be higher in patients with mutations in MSH2 as compared to patients with MLH1 mutations. Women with MSH6 mutations appear to be more likely to develop endometrial carcinoma. Other HNPCC-associated extracolonic neoplasms include carcinomas of the small bowel, pancreas, hepatobiliary tree, brain, and skin. The Muir–Torre syndrome, first described in 1967, refers to patients with HNPCC who also develop benign or malignant sebaceous skin neoplasms (sebaceous adenomas, carcinomas, squamous or basal cell), and multiple keratoacanthomas. The syndrome usually arises from mutations in MSH2. The development of glioblastoma multiforme, in association with HNPCC, is called Turcot’s syndrome. The Muir–Torre syndrome is also used to describe central nervous system neoplasms occurring in FAP, although these are usually medulloblastomas instead of glioblastomas. Approximately a third of patients with Turcot’s syndrome have mutations in one of the mismatch repair genes. HNPCC is associated with a 50% risk of a second carcinoma within 15 years of the initial carcinoma diagnosis 53 compared with 5% in the general population. Information regarding the cumulative risk of developing various HNPCC-associated malignancies has been summarized from several reports and tabulated in ▶ Table 21.6. 10 , 48 , 53 , 59 , 60
Plaschke et al 61 analyzed the involvement and phenotypic manifestations of MSH6 germline mutations in families suspected of HNPCC. Patients were preselected among 706 families by MSI, immunohistochemistry, and/or exclusion of MLH1 or MSH2 mutations and were subjected to MSH6 mutation analysis. Clinical and molecular data of MSH6 mutation families were compared with data from families with MLH1 and MSH2 mutations. They identified 27 families with 24 different pathogenic MSH6 germline mutations, representing 3.8% of the total of the families, and 14.7% of all families with DNA mismatch repair gene mutations. The median age of onset of colorectal carcinoma in putative mutation carriers was 10 years higher for MSH6 (54 years) compared with MLH1 and MSH2 (44 years). Relative to other malignancies, colorectal carcinoma was less frequent in MSH6 families compared with MLH1 and MSH2 families. In contrast, the frequency of non–HNPCC-associated neoplasms was increased. Later age of disease onset and lower incidence of colorectal carcinoma may contribute to a lower proportion of identified MSH6 mutations in families suspected of HNPCC. However, in approximately half of these families, at least one patient developed colorectal or endometrial carcinoma in the fourth decade of life. Therefore, a surveillance program as stringent as that for families with MLH1 or MSH2 mutations is recommended.
Approximately 60% of families that meet the Amsterdam-I criteria (HNPCC) have a hereditary abnormality in a DNA mismatch repair gene. Carcinoma incidence in Amsterdam criteria-I families with mismatch repair gene mutations is reported to be very high, but carcinoma incidence for individuals in Amsterdam criteria-I families with no evidence of a mismatch repair defect is unknown. Lindor et al 62 conducted a study to determine if carcinoma risks in Amsterdam criteria-I families with no apparent deficiency in DNA mismatch repair are different from carcinoma risks in Amsterdam criteria-I families with DNA mismatch repair abnormalities. 161 Amsterdam criteria-I pedigrees from multiple population- and clinic-based sources in North America and Germany were identified and grouped into families with (group A) or without (group B) mismatch repair deficiency by testing. A total of 3,422 relatives were included in the analysis. Group A families from both population- and clinic-based series showed increased incidence of HNPCC-related carcinomas. Group B families showed increased incidence only for colorectal carcinoma (standardized incidence ratio [SIR], 2.3) and to a lesser extent than group A (SIR, 6.1). Families who have fulfilled Amsterdam criteria-I but who have no evidence of DNA mismatch repair defect do not share the same carcinoma incidence as families with HNPCC with mismatch-repair deficiency. Relatives in such families have a lower incidence of colorectal carcinoma than those in families with HNPCC (Lynch syndrome) and incidence may not be increased for other carcinomas. These families should not be described or counseled as having HNPCC. To facilitate distinguishing these entities, the designation of “familial colorectal cancer type X” is suggested to describe this type of familial aggregation of colorectal carcinoma.
Jass 63 dispelled any thoughts that the morphogenetic pathway involved flat adenoma or de novo carcinoma in these cases. In a review of 131 carcinomas, none was small and superficial. Residual adenoma (contiguous with carcinoma) was present in 3 of 3 (100%) in situ carcinomas, 8 of 9 (89%) carcinomas involving only submucosa, 4 of 14 (29%) carcinomas limited to the muscle coat, and 13 of 105 (12%) carcinomas extending beyond the muscle coat. Lynch type II syndrome colon carcinomas show a significant increase in the proportion of mucinous, poorly differentiated carcinomas, and Crohn-like lymphoid reaction around the lesion as well as a higher rate of synchronous and metachronous carcinomas. 35 , 64
Other investigators have also studied HNPCC. Sankila et al, 65 compared the survival rates of 175 patients with hMLH1-associated HNPCC with those of 14,000 patients with sporadic colorectal carcinoma diagnosed at less than 65 years. The overall 5-year cumulative relative survival rate was 65% for patients with HNPCC and 44% for patients with sporadic colorectal carcinoma.
In a series of 1,042 Japanese patients who underwent resection for colorectal carcinoma, 3.7% were found to have HNPCC. 66 The characteristic early age of onset, right-sided predominance, and favorable survival were confirmed. Metachronous colorectal carcinoma developed significantly more often in cases with HNPCC (12.8 vs. 1.8%), and metachronous extracolonic malignancies also developed more often (10.2 vs. 3.5%). In cases with HNPCC, the mean interval between initial operation and the diagnosis of the second malignancy was 61 months (range, 12–153 months). These findings stress the importance of long-term follow-up.
Rodríguez-Bigas et al 67 reported the experience from the HNPCC Registry at Roswell Park in Buffalo, New York, which included 301 people in 40 families. In 284 of 301 people, 363 carcinomas were identified. Colorectal carcinomas alone were identified in 64% and in conjunction with extracolonic malignancies in another 11%. Extracolonic malignancies alone were identified in 25%. The median age at diagnosis of colorectal carcinoma was 48 years. Right-sided malignancies predominated (55%), synchronous and metachronous lesions were noted in 33%, and synchronous or metachronous adenomas were documented in 51% of those studied. Generational anticipation was also noted.
Hampel et al 68 assessed the frequency of the mismatch repair genes MLH1, MSH2, MSH6, and PMS2, in patients with a new diagnosis of colorectal carcinoma to identify patients with the Lynch syndrome. Genotyping of the carcinoma for MSI was the primary screening method. Among patients whose screening results were positive for MSI, they searched for germline mutations in the MLH1, MSH2, MSH6, and PMS2 genes with the use of immunohistochemical staining for mismatch repair proteins, genomic sequencing, and deletion studies. Family members of carriers of the mutations were counseled and those found to be at risk were offered mutation testing. Of 1,066 patients enrolled in this study, 19.5% had MSI, and 2.2% of these patients had a mutation causing the Lynch syndrome. Among the 23 probands with the Lynch syndrome, 10 were more than 50 years of age and 5 did not meet the Amsterdam criteria or the Bethesda guidelines for the diagnosis of HNPCC. In the families of 21 of the probands, 117 persons at risk were tested, and of these 52 had Lynch syndrome mutations. They concluded that routine molecular screening of patients with colorectal adenocarcinoma for the Lynch syndrome identified mutations in patients and their family members that otherwise would not have been detected. In most centers, it would not seem feasible to test all patients with colorectal carcinoma.
The characteristics of HNPCC mandate the development of surveillance programs. Screening recommendations have varied, but the recommendation by Lynch et al 69 for gene carriers has been colonoscopy, beginning at age 20 to 25 years or at least 5 years earlier than the earliest age at which colon carcinoma was diagnosed in a particular kindred with the procedure repeated every other year until the patient is 30 years of age and then annually thereafter. The frequency of colonoscopic examination is justified by the finding that HNPCC adenomas have repair deficient cells with rapidly and relentlessly accumulating mutations that support the clinical concept of “aggressive adenomas” and accelerate the adenoma–carcinoma sequence. The authors strongly believe that germline carriers should be offered prophylactic subtotal colectomy.
The importance of screening colonoscopy was underscored by the report of Sankila et al, 65 who compared the colorectal carcinoma death rates of two groups of HNPCC patients—those screened every 3 years and controls who refused screening. The authors’ data indicated that one carcinoma was prevented for every 2.8 polypectomies performed. This is in stark contrast to the National Polyp Study data for the general population, which gave a range of 41 to 119 polypectomies per carcinoma prevented.
The importance of screening is further emphasized by the study that compared screened with unscreened (by choice) “controls” evaluated over a 15-year period with 252 relatives at risk for HNPCC, 119 of whom declined screening. In the screened group, 8 of 133 (6%) developed colorectal carcinoma compared to 19 (16%) in the unscreened group. 70 de Vos tot Nederveen Cappel et al 71 examined the stage of the screening detected carcinomas in relation to the surveillance interval and to assess the risk of developing colorectal carcinoma while on the program in 114 families in the Dutch Family Registry. A total of 35 carcinomas were detected while on the program. With intervals between colorectal carcinoma and the preceding surveillance examination of 2 years or less, carcinomas were at Dukes’ stage A (n = 4), B (n = 11), and C (n = 1). With intervals of more than 2 years, carcinomas were Dukes’ stage A (n = 3), B (n = 10), and C (n = 6). The 10-year cumulative risk of developing colorectal carcinoma was 10.5% in proven mutation carriers, 15.7% after partial colectomy, and 3.4% after subtotal colectomy. There is a substantial risk of developing colorectal carcinoma while on the program. However, all carcinomas but one of subjects who underwent a surveillance examination 2 years or less before detection were at a local stage. They therefore recommend surveillance for HNPCC with an interval of 2 years or less.
For women who are gene carriers, an annual endometrial aspiration curettage should begin at age 30 years. Those patients should be advised of the option of ovarian carcinoma screening with transvaginal ovarian ultrasonography, Doppler color blood flow imagery, and CA-125 evaluation. They should be encouraged to have their children early so they can consider the option of undergoing prophylactic total abdominal hysterectomy and bilateral salpingo-oophorectomy between the ages of 35 and 40 years.
Screening for breast and gastric carcinoma should be initiated earlier in life than might otherwise be considered. If at least one family member is affected, gastroscopy beginning at age 30 to 35 years and every 1 to 2 years is advised. 53 Also, only if at least one family member is affected, ultrasonography of the urinary tract and urine cytology beginning at age 30 to 35 years and every 1 to 2 years is advised. 56
Mecklin and Jarvinen 72 analyzed 22 Finnish HNPCC families followed up for 7 years. Metachronous neoplasms were diagnosed in 41% of patients treated by segmental resection and 24% of those treated by subtotal colectomy. Extracolonic carcinoma was diagnosed in 30%. The most common malignancy was biliopancreatic carcinoma, which accounted for all five deaths related to carcinoma. The authors concluded that subtotal colectomy is superior to hemicolectomy or segmental resection in patients with HNPCC and that regular follow-up is necessary for surveillance of remaining bowel and extracolonic malignancies.
Itoh et al 73 conducted a study of 130 HNPCC kindreds to determine the risk of death from malignancy in first-degree relatives. The authors found a sevenfold increase in the risk of colon carcinoma in both sexes. In women relatives, the risk of breast carcinoma was increased fivefold, and a lifetime risk of breast carcinoma was 1 in 3.7. From these results, the authors recommended a screening program.
Because clinical premonitory signs such as multiple colonic polyps seen in cases of familial polyposis coli are lacking, the clinician must rely on the clinical findings and a family history typical of HNPCC. Disorders that have been considered in the differential diagnosis of HNPCC have included FAP, attenuated FAP, juvenile polyposis coli, and Peutz–Jeghers syndrome. 35 Once the diagnosis has been made, the physician is faced with the responsibility of informing the patient that other family members are at risk because the genetic implications for carcinoma expression are profound. Identification of individuals at risk may be aided by inspection of the patient’s skin, which may provide clues to the existence of a carcinoma associated with genodermatosis. Criteria providing clues to the diagnosis of Lynch type II syndrome include any patient presenting with an early onset of carcinoma of the colon (particularly of the proximal colon in the absence of multiple polyps), endometrium, or ovaries (particularly when the patient is younger than age 40 years) 1 ; any patient with multiple primary carcinomas in which the lesions are integral to Lynch type II syndrome (carcinoma of the colon, endometrium, or ovary and other adenocarcinomas) 2 ; and any patient who states that one or more first-degree relatives manifested early onset of the carcinomas integral to Lynch type II syndrome. 3
It is important to determine whether an HNPCC syndrome is present because the operative procedure of choice for the index carcinoma is subtotal colectomy as opposed to a more limited resection. 35 , 69 In the case of a woman who has completed her family, the resection may be extended to a prophylactic hysterectomy and bilateral salpingo-oophorectomy because of the patient’s inordinately high risk of development of carcinoma of the endometrium and ovaries.
The lifetime risk of endometrial and ovarian carcinomas in HNPCC is up to 60 and 12%, respectively. 74 Watson et al 75 collected data on 80 ovarian carcinoma patients who are members of HNPCC families, including 31 known mutation carriers, 35 presentive carriers (by colorectal/endometrial carcinomas status), and 14 at-risk family members. Among frankly epithelial cases, most carcinomas were well or moderately differentiated. Ovarian carcinoma in HNPCC differs from ovarian in the general population in several clinically important respects. It occurs at a markedly earlier age (42.7 years). It is more likely to be epithelial (95.6%). If it is a frankly invasive epithelial carcinoma, it is more likely to be well or moderately differentiated (85% were International Federation of Obstetrics and Gynecology [FIGO] stage I or II at diagnosis). HNPCC patients with ovarian carcinoma are more likely to have a synchronous endometrial carcinoma (21.5%).
Familial Colorectal Carcinoma
Ten to fifteen percent of patients with colorectal carcinoma and/or colorectal adenomas have other affected family members, but their family histories do not fit the criteria for either FAP or HNPCC and may not appear to follow a recognizable pattern of inheritance, such as autosomal-dominant inheritance. Such families are categorized as having familial colorectal carcinoma. The presence of colorectal carcinoma in more than one family member may be due to genetic factors, shared environmental risk factors or even to chance. 10 With a family history, the risk of colorectal carcinoma is increased earlier in life than later such that at age 45 years the annual incidence is more than three times higher than average-risk people, whereas at age 70, risk is not significantly different. The incidence in a 35- to 40-year-old is about the same as that of an average-risk person at age 50 years. A personal history of adenomatous polyps confers a 15 to 20% risk of subsequently developing polyps. A history of adenomatous polyps in a sibling or parent is also associated with increased risk of colorectal carcinoma. Expert recommendations on screening for such persons are similar to those with a positive family history of colorectal carcinoma. Most experts suggest that screening should begin at age 35 to 40 years when the magnitude of risk is comparable to that of a 50-year-old. Because the risk increases with extent of family history, there is room for clinical judgment in favor of even earlier screening, depending on the details of the family history. A common but unproven clinical practice is to initiate colorectal carcinoma screening 10 years before the age of the youngest colorectal carcinoma case in the family.
Evidence demonstrates that patients in families with breast and colon carcinoma (hereditary breast and colon carcinoma) may have a carcinoma family syndrome caused by CHEK2 1100delC mutations that are present in a subset of the families. 76 The CHEK2 mutation is incompletely penetrant.
Genetic Testing
Genetic testing provides the ability to determine who is and who is not at risk for disease before the onset of symptoms. Such information is becoming essential for proper management of patients and their families. In individuals who inherit mutant genes, simple preventive measures often can reduce morbidity and mortality and allow more thoughtful planning for the future. The benefits of genetic testing are equally important for those families who are found not to carry the relevant mutation; these individuals are spared unnecessary medical procedures and tremendous anxiety. However, genetic testing is not without its problems.
These problems can be broadly divided into psychological or technical in nature. From the societal view, issues related to insurance, employment, discrimination, and privacy have garnered much concern and attention. The technical challenges associated with genetic testing can be just as formidable and are often overlooked. Even when the mutated gene is known, routine genetic testing may fail to identify mutations. As a result of these uncertainties, genetic testing that fails to find the mutation is often inconclusive. Studies have shown that these inconclusive results may be misinterpreted by the patient and physicians and are a source of great anxiety. Because of the complex psychosocial and technical issues, it is clear that genetic testing should never be offered to patients without appropriate genetic counseling.
Prior to embarking upon any genetic testing, it is critically important that the individual at risk of developing carcinoma and the referring physician as well have an understanding of what the testing is designed to do. To this end, genetic counseling is of paramount importance for patients to fully understand the limitations of genetic testing and will aid in the management of patients who are susceptible to colorectal carcinoma. The management of individuals who may be at increased risk for an inherited colorectal carcinoma susceptibility is complex. Knowledge about genetic information can contribute to clinical management at several stages during patient care. Obtaining and interpreting genetic information can be a lengthy process but is essential to avoid harm and to heighten the benefits of genetic testing.
Genetic counseling and interpreting genetic test results can be complex but without knowing the limitations of the methods used and the lifetime probability of developing carcinoma in individuals who carry a gene that predisposes to carcinoma, misinterpretation may lead to false assurance. Wong et al 77 conducted a review of the literature and combined with their clinical and research experience suggested a guide to enable various health care providers to better counsel patients in their quest for advice on prevention, early detection, and surveillance for colorectal carcinoma. Much of the following information was extracted from their review.
Interpretation of genetic tests for colorectal carcinoma is not as straightforward as interpreting a blood sugar test to ascertain whether it is elevated, normal, or lowered. Genetic counseling is a dynamic communication process between the patient and the counselor who provides education and support within a multidisciplinary team. Counseling is targeted toward individuals who are interested in having a personal risk estimate. Those at greatest risk for developing malignancy may benefit by assessing current screening tests, whereas those at lower risk will be reassured that their risk for colorectal carcinoma is not as high as they thought.
Furthermore, survivors of malignancies may want to know their risk of recurrence or of a second primary neoplasm and the risk for their relatives, especially their children. Carcinoma is a genetic disease but is not necessarily inherited. Virtually all neoplasms and certainly all malignant ones result in part from genes that mutate somatically (during one’s lifetime). In contrast, a minority of neoplasms arises from an inherited gene that confers susceptibility in development. Genetic counseling is appropriate when the family history is suggestive of a heritable predisposition for malignancy. In general, the counseling process is composed of discrete elements: risk assessment, informative counseling, supportive counseling, and follow-up. Before initiating the process, it is important for the counselor to gain insight into the action of these components from the perspective of the patient. This is initially obtained by contracting. Contracting allows patients to know what to expect from genetic counseling and ensures their needs will be elicited and addressed by the counselor.
The presence or absence of a family history of benign and malignant neoplasms helps determine whether a person has an increased probability of having an inherited susceptibility for malignancy. Other familial traits can be characteristic signs of a genetic syndrome and suggestive that an increased risk for carcinoma may be present in a family. Pedigree analysis is multigenerational and includes details from both sides of the proband’s family. Current age or age at death is ascertained for everyone, because early death may explain the absence of a positive family history. Genetic counseling is recommended for individuals with an absolute risk greater than 10% (▶ Table 21.7) for developing carcinoma of the colon and rectum.
Providing risk assessment is only one part of the overall education process in genetic counseling. Explaining what genes are, how they are passed to subsequent generations, and the natural history of the neoplastic disease is an integral part of genetic counseling. Other educational aims include the recommendation or provision of screening modalities for early detection and preventive options. Medical intervention such as prophylactic surgery or chemoprevention, and the possible limitations of these treatments are issues for members of hereditary carcinoma families. Obtaining knowledge may empower the individual and family by dispelling the myths about malignancy and by providing guidelines that help in decision making. It also establishes the foundation of informed consent for genetic testing.
Indications
Genetic testing is discussed when the patient’s family history of carcinoma falls in the autosomal-dominant mode of inheritance. Appropriate situations include families who carry a mutation in APC, hMLH1, hMSH2, hPMS1, hPMS2, or hMSH6 or families fulfilling the clinical FAP or ICG-HNPCC (International Collaborative Group on Hereditary Non-Polyposis Colorectal Cancer) criteria. The decision to undergo genetic testing is a personal one based on informed consent. The first phase in determining whether genetic testing is appropriate is genetic counseling.
Among FAP families, who are candidates for genetic testing? The diagnosis of FAP is made because of the presence of polyps on examination of the presenting patient (proband or index case). In autosomal-dominant inheritance, on average, 50% of the patient’s first-degree relatives (parents, siblings, and offspring) will be at risk. As soon as the FAP diagnosis emerges, either before or after operation, the surgeon should recommend that all first-degree relatives be examined. If a mutation in APC is identified in the patient’s germline DNA, the immediate family and other branches of the family can be encouraged to come forward for genetic counseling and to know their risk status if so desired. If a mutation is not detected, further genetic testing for APC mutations in the family is contraindicated and the clinical decision is to monitor first-degree relatives by colonoscopy at recommended intervals. Depending on the expression of colorectal carcinoma in the proband and the pattern of colorectal carcinoma and other malignancies in the family, genetic testing for HNPCC may be advisable. In every case of FAP, a genetic test to pinpoint the heritable, germline APC mutation is justified, with informed consent of the patient. As far as testing the patient’s family is concerned, a common exception (occurring in 30% of probands) is a patient with FAP whose parents are negative on endoscopic examination and who therefore has a “new mutation” in the APC gene. In this instance, the risk for colorectal carcinoma for siblings falls to that of the general population. Nonetheless, children of the patient will require testing (if a mutation was identified in the proband) if so desired, because they are at 50% risk for FAP. The only obvious example in which genetic testing is not recommended would be in an isolated patient with FAP with no living first-degree relatives.
What patients with suspected HNPCC should be referred for genetic counseling and testing? Because HNPCC is not always obvious based on clinical findings, either preoperatively or during resection, the clinical management of a possible HNPCC patient is complex. A detailed family history, with sites of malignancy verified, is critical to determine whether the patient fits the Amsterdam criteria and other associated features of HNPCC. Analysis of neoplastic material would provide assistance in determining whether to test the proband’s germline DNA (i.e., a blood sample) for HNPCC mutations (after genetic counseling) and refer the first-degree relatives should a mutation be detected. In the instance of a positive family history, it is ideal for testing to be conducted despite MSI phenotype. The clinical algorithm would be much the same as for FAP, with the exception that the multiple genes might need to be analyzed unless tests on pathogenic samples narrow the search. It is important to point out that at present, detection of a confirmed pathogenic mutation occurs in less than 50% of HNPCC families. In the absence of an identified deleterious mutation, genetic testing is inconclusive, which must be explained to the patient by an individual skilled in genetic counseling. Family members can be given general advice for colorectal carcinoma screening of first-degree relatives of patients with this malignancy.
Techniques
The molecular techniques used in testing for germline mutations in hereditary colorectal carcinoma have been described in detail by Rabelo et al 78 and the following is a synopsis of that work.
Germline DNA can be tested in three ways:
With in vitro–synthesized protein (IVSP) assay (also called protein truncation test or in vitro truncation test), specific gene segments are amplified by polymerase chain reaction (PCR) or reversed transcription (RT) PCR, transcribed and translated in vitro, and the protein product is analyzed by gel electrophoresis. If the amplified segment has a mutation that causes the production of a truncated protein, its smaller size will allow it to be more mobile in the gel.
Single-stranded conformation analysis (SSCA) is also a mutation detection technique in which specific gene segments are amplified by PCR or RT-PCR, denatured to separate the stands of the DNA product and analyzed by gel electrophoresis. The mutant DNA often migrates differently under certain conditions, allowing the putative localization of mutation within the segment.
DNA sequencing is the criterion standard technique for mutation detection, with up to 99% accuracy. It allows the precise identification of the mutation in the DNA sequence by pinpointing any change in the number or identity of bases.
Pathologic specimens are examined in two ways:
A clue to identify colorectal carcinoma caused by HNPCC is by an associated alteration in the length stability of repetitive tract DNA in the carcinoma of an affected individual. This is known as MSI, also called RER-positive phenotype. The presence of MSI + thereby increases the likelihood that direct testing for defects in HNPCC genes will be informative. There are three outcomes of MSI analysis. 56
If at least two markers tested show instability, the result indicates an increased likelihood that the colorectal carcinoma arose due to an HNPCC-associated mutation (MSI-H).
If no marker shows instability in the absence of a compelling family history, the result is usually interpreted as ruling out HNPCC (MSS stable).
If instability is seen in only one of the markers, the result does not indicate HNPCC. The clinical behavior of MSI-L carcinomas is identical to those with MSS, and does not have the mutational fingerprints of the clinical behavior of MSI-H carcinomas. MSI-L results occur as commonly as do MSI-H results.
Immunohistochemistry is a technique for diagnosing a carcinoma that lacks expression of a particular HNPCC gene. It examines either hMLH1 or hMSH2 protein expression in carcinomas by immunostaining using monoclonal antibodies. Formalin-fixed, paraffin-embedded adenocarcinoma tissue is the sample required for testing with a region of normal mucosa adjacent to the carcinoma for comparison. The absence of hMLH1 or hMSH2 immunostaining shows which gene is defective and should be analyzed for the presence of a germline mutation. Studies have shown that the lack of hMLH1 or hMLH2 immunostaining was associated with the presence of MSI+.
Genetic Testing for Familial Adenomatous Polyposis
Presymptomatic genetic diagnosis of FAP in at-risk individuals has been feasible with linkage and direct detection of APC mutations. 10 If one were to use linkage analysis to identify gene carriers, ancillary family members, including more than one affected individual, would need to be studied. With direct detection, fewer family members’ blood samples are required than for linkage analysis, but the specific mutation must be identified in at least one affected person by DNA mutation analysis or sequencing. Because approximately 96% of the mutations in FAP lead to a truncated protein, it has become routine to use IVSP assay (sometimes called protein truncation test) for mutation detection. When a truncated protein is identified, it is possible to localize the mutation to a specific segment of the gene and then use DNA sequencing to determine the mutated nucleotide(s). The use of IVSP as the sole genetic test in FAP misses approximately 20% of APC mutations. Another screening technique is based on analysis of electrophoretic migration of small segments of the wild-type mutant gene (SSCA). The sequential use of two molecular diagnostic tests has become a common practice: a simple and less expensive screening technique (of high sensitivity and moderate specificity), followed by a definitive test of high sensitivity, usually DNA sequencing.
These mutation search methods, APC protein truncation testing (from lymphocyte RNA), considerably enhance the feasibility of testing at-risk individuals without requiring DNA from multiple affected family members (as linkage requires). In particular, it is useful for testing in small families or in patients with spontaneous or “de novo” mutations (the first occurrence of FAP in a kindred), which may account for as much as one third of incident cases. 10 Only about 80% of APC mutations can be detected by this method. Therefore, the clinician cannot rule out FAP on the basis of this molecular test alone if other criteria support the FAP diagnosis. When the mutation in a family is known from one or more affected members, one test, usually IVSP, need be performed, as the expression pattern of the mutation is already established for that family. A positive result in such a test is considered a “mutation-positive” result, and the patient can be counseled as recommended. When the mutation in the family is not known, IVSP is performed. In the majority of cases, a mutation will be found in the APC gene that can be further characterized by DNA sequencing. Again, a “mutation-positive” result is obtained leading to the appropriate genetic counseling for the patient. Other options include DNA sequencing and linkage analysis. Even by combining two or more techniques, it is not possible to achieve 100% sensitivity, because the mutations may not be in the coding region of the gene or because a few FAP kindreds do not exhibit linkage to chromosome 5q. In such case, a “no mutation detected” result must not be interpreted as a “negative test” with the very important considerations for counseling the patient.
For at-risk individuals who have been found to be definitively mutation negative by genetic testing, there is no clear consensus on the need for or frequency of colon screening, though it would seem prudent that at least one flexible sigmoidoscopy or colonoscopy examination should be performed in early adulthood (age 18–25 years). 10
Molecular Genetic Diagnosis of Hereditary Nonpolyposis Colorectal Carcinoma
The HNPCC syndrome is more complex in terms of genetic testing because at least six genes involved in DNA mismatch repair predispose to this syndrome. The two most commonly involved genes are hMSH2 (involved in approximately 45% of cases) and hMLH1 (involved in approximately 49% of cases). Protein truncation is observed in more than 80% of HNPCC cases caused by mutations in hMSH2 and to a lesser degree in hMHL1. Currently, IVSP or SSCA or both are used, followed by DNA sequencing for precise characterization. In the case of a mutation already known in an affected member of the kindred, the same technique used to detect that mutation can be used, and a “mutation-positive” result leads to genetic counseling. If no mutation has been previously described in the kindred, analysis of hMLH1 and hMSH2 in an affected individual by IVSP or SSCA followed by DNA sequencing for confirmation is indicated. If a truncating mutation is found, a causative role can be inferred for the mutation. This “mutation-positive” result allows counseling of the affected individual and further testing of the kindred. If a nontruncating mutation is found, tests such as linkage analysis and functional assays may be required to discriminate between an “inconclusive” and a “mutation-positive” result. It is estimated that approximately 30% of mutations will be missed if IVSP is used as the sole genetic test and that a similar or higher percentage of false negatives may also be expected if SSCA is used instead. Again, even by combining two or more techniques, it is not possible to achieve 100% sensitivity. Other genes may be responsible (locus heterogeneity). A “no mutation detected” result must not be interpreted as a “negative test” result.
Summary
A proposed algorithm for genetic testing of individuals in FAP and HNPCC kindreds is given below. First, use IVSP to locate the mutation, then use DNA sequencing to characterize it precisely in the first affected family member. If the IVSP test is negative, DNA sequencing of the APC gene is warranted. When the mutation is defined for that kindred, the testing of other family members at risk may be accomplished by using IVSP alone. If the mutation in the first affected individual was identified by DNA sequencing, it is logical to continue to use this method for genetic testing of at-risk individuals. Considering the weighty psychological, ethical, and legal implications of the results of genetic tests, the use of two different techniques, one to detect the mutation and the second to identify and confirm it, is recommended in all cases. DNA sequencing, the most sensitive method for mutation detection, should be one of the techniques used. For HNPCC, two techniques are appropriate for initial testing of mutations in these genes, IVSP and SSCA. Screening with IVSP is recommended, but this preference may vary among laboratories. If a mutation is detected by any of these methods, DNA sequencing should be used to identify it precisely in the first affected member of a kindred. If these screening techniques are negative, the sequencing of both hMSH2 and hMLH1 may be warranted. If still negative, in view of the locus heterogeneity seen in HNPCC, the same screening protocol may have to be used for hPMS2, hPMS1, and hMSH6. Again, the use of two different tests in all cases is recommended and DNA sequencing is used and when the pathogenic mutation is defined for that kindred, the testing of other family members at risk may be accomplished with either IVSP or SSCA alone.
When a mutation (a variant gene) is identified by any of the methods, it must be validated as a pathogenic mutation (true-positive result) instead of a polymorphism, a common variant in the protein that does not contribute to the disease phenotype (false positive). When a mutation is not identified (“no mutation detected”), the negative result may be a “true negative” (no predisposing mutations in any relevant genes), or a “false negative” (undetected mutation may exist in known or unknown genes). If the person is affected with colorectal carcinoma, this is either a false negative in a familial colorectal carcinoma syndrome or a sporadic case. This “no mutation detected” result is the one most likely to be misinterpreted, leading to inappropriate genetic counseling. The use of two genetic tests decreases the rate of false-negative results. DNA sequencing is the most sensitive technique for this purpose and its use is advocated whenever a negative result is obtained by IVSP or SSCP for affected members with a strong family history. Even if the APC gene or all five DNA mismatch repair genes are fully sequenced, there will still be a residue of false-negative results caused by mutations in the noncoding regions of those genes, locus heterogeneity, or in still-unidentified genes.
A “negative test” in patients at risk for FAP or HNPCC will rule out these disorders only if a mutation has been identified in an affected family member. If tests to identify a mutation in an affected family member are negative, a “no mutation detected” result should be considered as noninformative or inconclusive (as if no test had been performed). The correct interpretation of a negative result in mutation analysis of hereditary colorectal carcinoma syndromes is mandatory to avoid adverse outcomes, because a false-negative result may lead to lack of appropriate endoscopic surveillance. Molecular genetic testing must be coupled with appropriate genetic counseling.
Interpretation of Genetic Test Results
Familial Adenomatous Polyposis
A summary for interpreting APC genetic test results is as follows. The implication of a mutation-positive test result is that APC mutation carriers need to be informed that prophylactic colectomy is necessary when adenomatous polyps become evident. Mutation carriers require surveillance by gastroscopy for extracolonic neoplasms in the upper gastrointestinal tract. Other variant manifestations in FAP include osteomas, cutaneous cysts, and congenital hypertrophy of retinal pigment epithelium (CHRPE). When a mutation has been identified in the family, direct gene testing of relatives who have not yet been clinically assessed will distinguish between those who carry the mutation and those who do not. About 30% of FAP cases are caused by new mutations in the APC gene. In these cases, the parents will not carry this mutation and are not at risk of FAP; only descendants of the proband are at 50% risk. What are the implications of a “negative test” result versus “no mutation detected” result? A negative test result is given if the patient does not carry the mutation that is known to exist in their family. In this instance, family members are not at increased risk for developing colorectal carcinoma compared with the general population and should follow guidelines for carcinoma surveillance for that group. If the patient is affected with FAP and complete coding sequence analysis of the APC gene fails to identify a mutation, this could mean that the APC gene is not responsible for the patient’s diagnosis. In this case, a “no mutation detected” result, APC gene testing has no predictive value for asymptomatic at-risk relatives. In these families, first-degree relatives should continue colorectal carcinoma surveillance annually between the ages of 12 and 25 years, every other year between the ages of 25 and 35 years, and every third year between 35 and 50 years. Family members who have not developed multiple adenomatous polyps on complete bowel examination by the age of 50 years are assumed to be unaffected by FAP. If a “no mutations detected” result occurs when an unaffected family member from an APC kindred is tested without testing an affected family member, it is important that the patient not be falsely reassured because other mechanisms may inactivate the APC gene or other genes may be involved.
APC I1307K
A mutation in the APC gene termed I1307K was found in about 6% of people of Ashkenazi Jewish descent and about 28% of Ashkenazim with a family history of colorectal carcinoma. It appears to be associated with an approximately twofold increase in colorectal carcinoma risk. 13 This alteration is a transversion of a single base from thymidine to adenine in codon 1307 of the APC gene. Analysis for this mutation is done by allele specific oligonucleotide analysis (extremely sensitive and specific) and consequently delivers a conclusive positive or negative test result. Genetic testing for this alteration is possible, but the clinical utility of such testing is uncertain. The mean age at which colorectal carcinoma occurs in people carrying the I1307K alteration is not known, nor has the natural history of colorectal carcinoma in carriers of I1307K been assessed or compared to sporadic colorectal carcinomas. No screening outcomes have been assessed in carriers of I1307K. Therefore, it is not yet known whether the I1307K carrier state should guide decisions about the age at which screening is initiated, the optimal screening strategy, or the optimal screening interval. 10
Hereditary Nonpolyposis Colorectal Carcinoma
A summary of interpreting HNPCC genetic testing results is given below. The implication of a positive mutation result is that individuals carrying a mutation in a gene that predisposes to HNPCC should be educated on the risk of colorectal carcinoma as well as carcinoma in other sites: endometrium, stomach, small bowel, biliary tract, ovary, pancreas, renal pelvis, and ureter. The risk to age 70 years for developing any neoplasm may be as high as 90% for males and 70% for females. 79
For mutation carriers who develop colorectal carcinoma, total abdominal colectomy with ileorectal anastomosis is recommended because the entire colonic mucosa is at risk for malignancy. Proctocolectomy may also be a consideration, because the distal rectum is also a possible site of carcinoma. Prophylactic resection may be an option for mutation carriers unwilling to undergo surveillance or when endoscopic polypectomy is difficult. It should be emphasized that there are no data on whether resection is effective in reducing overall mortality. All first-degree relatives of patients who carry a mutation in one of the mismatch repair genes should be counseled that they have a 50% chance of carrying the same mutation.
What are the implications of a negative test result versus no mutation detected? A patient who tests negative for a known family mutation is not at risk for HNPCC, because it is a conclusive negative test result. Subsequent carcinoma surveillance should be as for the general population. If a mutation in a family with strong circumstantial evidence for HNPCC has not been identified, the interpretation of a “no mutation detected” test result in the HNPCC-associated gene or genes tested is complex. It may be necessary to perform more than one screening test to strengthen the suspicion that a mutation in a mismatch repair gene exists in the family. The difference between a negative test result and a “no mutation detected” result with its subsequent implications is dependent on whether a mutation has been previously identified in the family in HNPCC families. If the person is affected with HNPCC, and no mutation was detected in hMLH1 and hMSH2 with IVSP assay and single-strand conformation polymorphism, other investigations may be offered to determine whether the patient carries a mutation in a mismatch repair gene. For example, testing for MSI in neoplastic tissue may be a worthy investment before sequencing hMLH1 and hMSH2. If no abnormality in hMLH1 and hMSH2 is found by sequencing, analysis of the rarer HNPCC-causing genes is indicated.
Caution should be exercised in providing genetic risk assessment on the basis of currently used germline mutation detection strategies. While screening for hMSH2 gene mutations in HNPCC kindreds, Xia et al 80 observed that using RT-PCR and the protein truncation test, the hMSH2 exon 13 deletion variant was found in more than 90% of individuals. This may lead to a misdiagnosis of HNPCC and has profound implications for counseling and genetic risk assessments of other family members.
Impact of Genetic Testing
Individuals need to know the implications and possible impact of genetic test results before testing. An ambiguous test result may be more distressing than a positive test result. Moreover, genetic testing often yields results that are probabilistic. The psychological burden of knowing that one is at high risk for developing a neoplasm may outweigh the possible benefits from intervention. Results of a study by Keller et al 81 suggest that expressed intention and attitude toward genetic testing do not reliably predict actual uptake of counseling or testing. The actual uptake of genetic testing for HNPCC in a clinical sample of 140 patients fulfilling clinical criteria of HNPCC was 26%. Some 60% of participants experienced pronounced distress related to their potential inheritance of the disorder compared to 35% among nonparticipants. Distress reached a clinically significant level in 28% of participants. Restricted communication within the family was observed frequently. Irrespective of groups, a positive attitude toward obtaining a gene test result predominated. Therefore, the benefits and risks of participating in genetic tests must be described before testing is begun. Undergoing early detection of colorectal carcinoma or prophylactic operation or both can save lives. Surveillance or operation can be targeted to specific sites that are more prone to neoplasia, depending on the identity of the gene. For instance, female members of HNPCC families have a 10-fold increased risk for developing endometrial carcinoma, with an age of onset 10 to 15 years earlier than the same disease in the general population. Options for intervention and early detection and provision of knowledge to other family members are common reasons why individuals seek testing for genes predisposing to carcinoma of the colon and rectum.
Gritz et al 82 examined the impact of HNPCC genetic test results on psychological outcomes among carcinoma-affected and carcinoma-unaffected participants up to 1 year after results disclosure. A total of 155 persons completed study measures before HNPCC genetic testing, and 2 weeks and 6 and 12 months after disclosure of test results. Mean scores on all outcome measures remained stable and within normal limits for carcinoma-affected participants, regardless of mutation status. Among unaffected carriers of HNPCC-predisposing mutations, mean depression, state anxiety, and carcinoma worries scores increased from baseline to 2 weeks post disclosure and decreased from 2 weeks to 6 months post disclosure. Among unaffected noncarriers, mean depression and anxiety scores did not differ, but carcinoma worries scores decreased during the same time period. Affected and unaffected carriers had higher mean test-specific distress scores at 2 weeks post disclosure compared with noncarriers in their respective groups; scores decreased for affected carriers and all unaffected participants from 2 weeks to 12 months post disclosure. Classification of participants into high-versus low-distress clusters using mean scores on baseline psychological measures predicted significantly higher or lower follow-up scores, respectively, on depression, state anxiety, quality of life, and test-specific distress measures, regardless of mutation status. Although HNPCC genetic testing does not result in long-term adverse psychological outcomes, unaffected mutation carriers may experience increased distress during the immediate postdisclosure time period. Furthermore, those with higher levels of baseline mood disturbance, lower quality of life, and lower social support may be at risk for both short- and long-term increased distress.
Examining a person’s DNA has the potential for far-reaching consequences beyond the person being tested. Genetic testing can reveal information about relatives with whom we share a common genetic legacy. Despite the possible benefits of testing for susceptibility for malignancy, the uncertainty and limitations of current efforts to lower mortality or morbidity contribute to the psychosocial and ethical complexities. Genetic information must remain confidential. However, breach of confidentiality has been considered permissible if all of the following conditions are met: reasonable effort to encourage disclosure has failed, harm is likely to occur if the information is withheld, the harm is serious and avoidable, and the disease is treatable or preventable. 83 Conflict between issues of privacy and duty to forewarn family members arises because genetic information is, at once, individual and familial. Several key issues may be raised in genetic testing. First, at times genetic test results need to be interpreted in the context of the results of other family members. Second, family members should be independently and autonomously counseled. Third, the decision of each member regarding testing should be followed without coercion by other family members. Fourth, genetic test results have familial implications whether results are disclosed to other family members or not. 84
To provide information useful in the education and counseling in individuals considering genetic testing, Lerman et al 84 conducted structured interviews with 45 first-degree relatives of colorectal carcinoma patients. Fifty-one percent of respondents indicated that they definitely would want to obtain a genetic test for colon carcinoma susceptibility when it is available. Motivation for genetic testing included the following: to know if more screening tests are needed, to learn if one’s children are at risk, and to be reassured. Barriers to testing included concerns about insurance, test accuracy, and how one’s family would react emotionally. Most participants anticipated that they would become depressed and anxious if they tested positive for a mutation; many would feel guilty and still worry if they tested negative. These preliminary results underscore the importance of the potential risks, benefits, and limitations of genetic testing with particular emphasis on the possibility of adverse psychological effects and implications for health insurance. Ethical issues of revealing or concealing genetic information will be debated long into the future because the potentially enormous consequences must be carefully considered. Hadley et al 85 assessed the impact of genetic counseling and testing on the use of endoscopic screening procedures and adherence to recommended endoscopic screening guidelines in 56 symptomatic at-risk individuals in families known to carry an HNPCC mutation. They analyzed data on colonoscopy and flexible sigmoidoscopy screenings collected before genetic counseling and testing and 6 and 12 months postgenetic counseling and testing on 17 mutation-positive and 39 true-negative mutation individuals. Among mutation-negative individuals, use of colonoscopy and flexible sigmoidoscopy decreased significantly between pre- and postgenetic counseling and testing. Among mutation-positive individuals, a nonsignificant increase in use was noted. Age was also associated with use of endoscopic screening after genetic counseling and testing. More mutation-negative individuals strictly adhered to guidelines than did mutation-positive individuals (87 vs. 65%). They concluded genetic counseling and testing for HNPCC significantly influences the use of colonic endoscopy and adherence to recommendations for colon carcinoma screening.
Patients considering genetic testing need to be informed about the potential for genetic discrimination. This is of great concern for asymptomatic family members, because disclosure of a positive mutation result to third parties could be used to limit, raise rates for, or deny access to individual health insurance. However, nondisclosure could nullify a patient’s insurance contract.
A survey of medical directors of United States life insurance companies indicated that familial colon or breast carcinoma constituted sufficient grounds to deny insurance (1 of the 27) or charge higher premiums (6 of the 27), despite not having actuarial data to support underwriting (calculating premiums) guidelines pertaining to these carcinomas.
In the United States, several states have safeguards against the potential misuse of genetic information. The potential for misuse of genetic information has led to federal initiatives in the United States to regulate the use of genetic information. In the context of insurance and employment, it is unclear whether the Americans with Disabilities Act of 1990 (ADA) will provide adequate protection to carriers of carcinoma-susceptibility genes, because the Equal Employment Opportunity Commission does not view a person with genetic predisposition for a disease or a carrier of a gene for a late-onset disorder as having a disability. This implies that these persons are not protected under the ADA. Legislation has been introduced to extend the definition of disability to “genetic or medically identified potential of or predisposition toward a physical or mental impairment that substantially limits a major life activity.” For now, the ADA has been interpreted to offer protection only to those who develop symptoms.
Health Insurance Portability and Accountability Act (Kennedy–Kassebaum bill) came into effect in the summer of 1997. It defined genetic information as part of an individual’s health status. This act was implemented to prohibit employers and insurers from excluding individuals in a group from coverage or charging higher premiums on the basis of health status. Also in 1997, the Genetic Information Nondiscrimination in Health Insurance Act (Slaughter–Snowe bill) called for a ban of the use of “genetic information” in denying or setting rates for group health insurance. In this act, genetic information was defined broadly to include genetic tests as well as information about inherited features. This bill called for a limit on the collection or disclosure of genetic information without the written consent of the individual.
Fourteen states have enacted legislation to provide safeguards and to protect misuse of genetic information by insurers and employers. Other states have followed suit, and amendments are being made to those existing laws in some states, as reviewed by Offit. 86
In summary, individuals undergoing genetic testing should understand the advantages and disadvantages of receiving and sharing genetic results. Attempting to protect the individual from genetic discrimination by disseminating results to only a few selected medical staff could impede care. So far, there has been no substantial use of genetic information by health insurers, and laws restricting its use have thus far maintained social fairness in the area of health insurance. When integrated with existing testing protocols for colorectal carcinoma and when applied with appropriate caveats particularly regarding interpretation of negative results, genetic testing can result in improved management of patients and families.
Alternate Pathway of Carcinogenesis
An understanding of the mechanisms that explain the initiation and early evolution of colorectal carcinoma should facilitate the development of new approaches to effective prevention and intervention. Jass et al 87 have proposed a model for colorectal neoplasia in which APC mutation is not placed at the point of initiation. Other genes implicated in the regulation of apoptosis and DNA repair may underlie the early development of colorectal carcinoma. Inactivation of these genes may occur not by mutation or loss but through silencing mediated by methylation of the gene’s promoter region. hMLH1 and MGMT are examples of DNA repair genes that are silenced by methylation. Loss of expression of hMLH1 and MGMT protein has been demonstrated immunohistochemically in serrated polyps. Multiple lines of evidence point to a “serrated” pathway of neoplasia that is driven by inhibition of apoptosis and the subsequent inactivation of DNA repair genes by promoter methylation. The earliest lesions in this pathway are aberrant crypt foci (ACF). These may develop into hyperplastic polyps or transform while still of microscopic size into admixed polyps, serrated adenomas, or traditional adenomas. Carcinomas developing from these lesions may show high- or low-level MSI (MSI-H and MSI-L, respectively) or may be microsatellite stable (MSS). The suggested clinical model for this alternative pathway is the condition hyperplastic polyposis. Hyperplastic polyposis presents a plausible model for the following reasons:
Polyps in this condition may show MSI and silencing of relevant DNA repair genes including hMLH1.
Methylation is demonstrated in DNA extracted from hyperplastic polyps in a subset of subjects with hyperplastic polyposis. The finding of methylation is concordant within multiple polyps in such cases, whereas discordant findings occur in subjects with multiple adenomas.
The requisite plasticity in methylator pathways is evident in hyperplastic polyposis in which all types of epithelial polyp may occur (hyperplastic, admixed, serrated adenoma, and traditional adenoma), and carcinomas may be MSI-H, MSI-L, or MSS (even within the same subject).
The condition hyperplastic polyposis may be familial. Jass et al 87 believe that molecular and morphologic observations have stripped the hyperplastic polyp of its long-presumed innocence. However, the fact that the vast majority of hyperplastic polyps will never progress to carcinoma has not altered. It is impractical to advocate the removal of every minute hyperplastic lesion. On the other hand, more attention might be given to subjects with “high-risk” hyperplastic polyps. High-risk features would include multiplicity (more than 20), size (greater than 10 mm), proximal location, associated polyps with dysplasia, and a family history of colorectal carcinoma. New diagnostic criteria and markers are required to distinguish innocent hyperplastic polyps from their serrated counterparts with a malignant potential that belies their deceptively bland morphology.
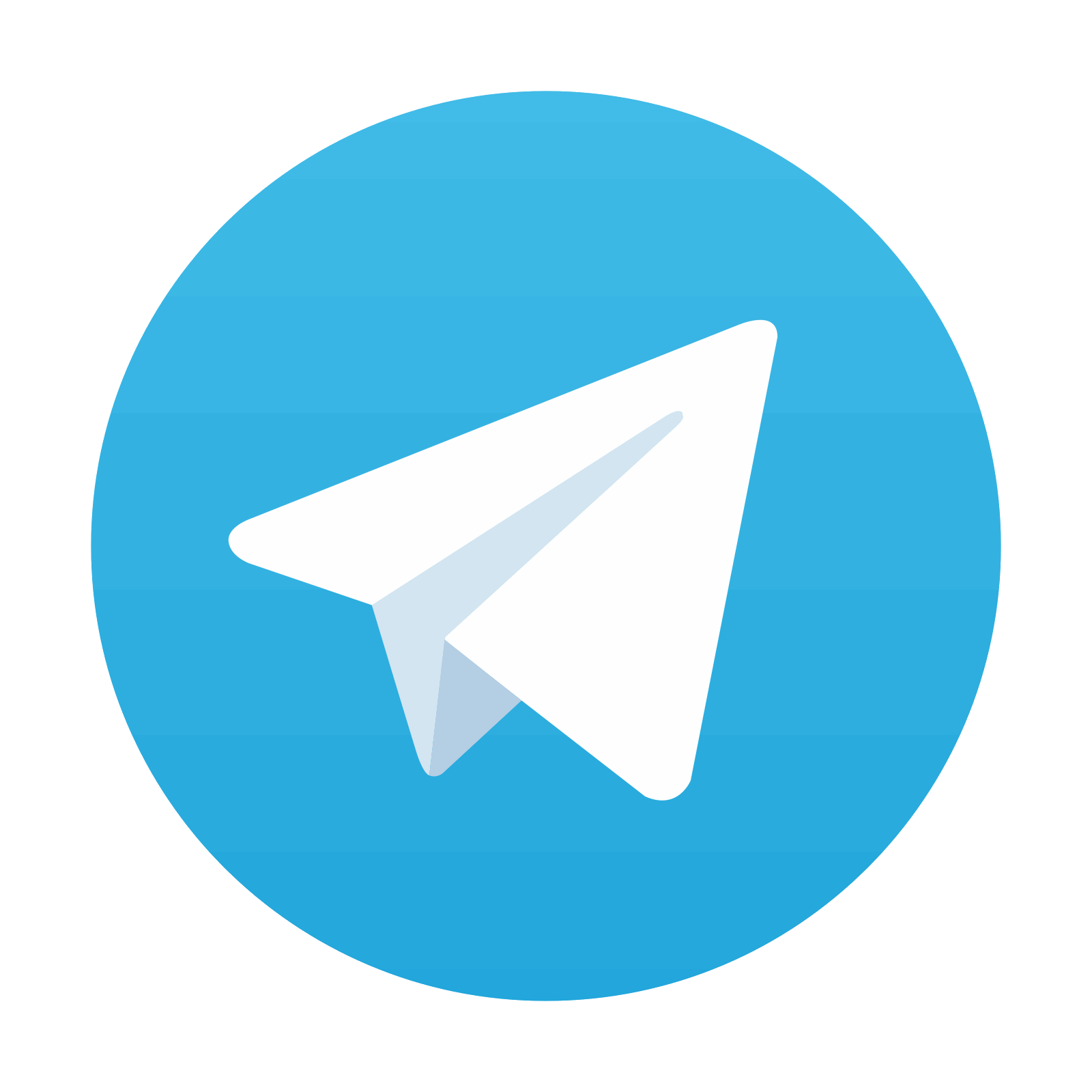
Stay updated, free articles. Join our Telegram channel

Full access? Get Clinical Tree
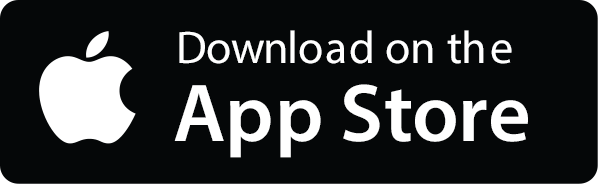
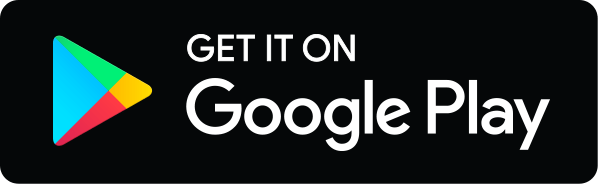
