Obesity is an emerging global epidemic with profound challenges to world health care economies and societies. Traditional approaches to fighting obesity have not shown promise in promoting a decline in obesity prevalence. The gut microbiota is becoming widely appreciated for its role in regulating metabolism and thus represents a target for new therapies to combat obesity and associated comorbidities. This article provides an overview of altered microbial community structure in obesity, dietary impact on the gut microbiota, host-microbe interactions contributing to the disease, and improvements in microbial assemblage after bariatric surgery and with therapies targeting the gut microbiome.
Key points
- •
Shifts in the gut microbiome are inseparably associated with the development of obesity and comorbidities.
- •
Transfer of dysbiotic microbial communities confers disease phenotypes in recipients, supporting a central role for microbe-mediated regulation of metabolism.
- •
Bariatric surgery, the most effective treatment of morbid obesity, results in rapid changes in the gut microbiota, with concurrent improvements in metabolic parameters.
- •
Deeper understanding of host-microbe interactions may hold promise in the treatment of obesity, which remains a global epidemic.
Introduction: obesity and the gut microbiota
The increase of obesity and its related comorbidities in westernized countries over the past 4 decades presents an emerging global epidemic with profound challenges to world health care economies and societies. In the past 35 years, the rate of adult obesity has risen by 75% globally. This number is greater among children. Stratified assessment of body mass index further shows disproportionate increases among the most severely obese (≥35 kg/m 2 ), compared with the lesser obese (≥30 kg/m 2 ), showing the scale of the problem. However, obesity and its comorbidities, including metabolic syndrome, diabetes, and heart disease, have detrimental effects on quality of life and substantial costs to individuals and societies. Thus, the need for understanding the complexity of pathophysiologic events and elucidating effective interventions remain urgent.
The cause of obesity is multifactorial, including the complex interaction of genetics and environment, which encompasses diet, developmental factors, lifestyle (eg, hedonistic tendencies, altered sleep patterns), and antibiotic use. Intestinal microbes are affected by all of these factors in their community structure and function, and in turn initiate host-microbe interactions that may disrupt metabolic and immune homeostasis. Fecal microbiota transplant (FMT) of microbes under environmental stressors, like diet and obesity, can induce a similar phenotype in recipients. The gut microbiome is by definition a microbial organ (vital to intestinal and systemic functions), and one that people cannot live without, but is also an organ that is transplantable (ie, via FMT). This technique is commonly used for Clostridium difficile infection and has only recently been studied for use in other conditions, including obesity. However, other therapies targeting the microbiome, such as prebiotics and probiotics, may confer modest, but positive, improvements for symptoms associated with obesity and its comorbidities.
Although an extreme measure reserved for the morbidly obese, one of the most effective strategies to decrease obesity is bariatric surgery, which profoundly changes the gut microbiota and energy balance, and alters physiologic and endocrine metabolic states. It is expected that by changing metabolic set points, desired weight can be achieved. Therefore, understanding of the mechanisms behind bariatric surgery and associated changes in the gut microbiota may be leveraged to develop new therapies to fight the obesity epidemic. This article explores these concepts by providing an overview of altered microbial structure and function in obesity, host-microbe interactions driving obesity, dietary influence on the microbiome, improvements in metabolism and microbial structure with Roux-en-Y gastric bypass (RYGB), the host-microbe interactions driving obesity, and current therapies targeting the gut microbiome to facilitate positive metabolic outcomes.
Paradigms in gut microbiota during obesity
Obesity-Driven Alterations in Gut Microbiota
The human body contains huge numbers of microbes, including thousands of bacterial species, in addition to many eukaryotes, Achaea, protists, and viruses, which collectively contain an estimated 5 million genes that have profound metabolic and immunomodulatory effects on their mammalian hosts. The community of microbes is termed the microbiota, whereas their collective genes are called the microbiome. Both the state of obesity and westernized diets are associated with microbial dysbiosis, which is a deviation from microbial organization that would otherwise promote optimal metabolic homeostasis. Dysbiotic microbiota in obesity is characterized by decreased diversity in the microbial community and by an increased ratio of the phylum Firmicutes to the phylum Bacteroidetes. The change in the Firmicutes/Bacteroidetes ratio occurs in both mice and humans, and weight loss restores microbial composition. Note that 3 genera of bacteria are often overrepresented in obesity in humans: Bacteroides and Prevotella (both Bacteroidetes) and Ruminococcus (Firmicutes). In addition to composition, major functional differences are observed in metabolic capacity of the microbial community. For instance, decreases in short-chain fatty acid (SCFA) producers, such as from the phylum Actinobacteria and blooms in pathogenic bacteria from the phylum Proteobacteria, occur in obesity. In addition to bacteria, recent work shows that the microbiota metabolic networks include yeast and archaea, which synergistically produce and use metabolites collectively with bacteria. Although this area is still underexplored, recent work suggests that yeast species abundance is lower in obesity, and supplementation with Saccharomyces cerevisiae improves metabolic parameters and adiposity.
When dysbiotic communities of bacteria are transferred to naive germ-free (GF) mice, the recipient mice develop increased adiposity, showing a direct impact of the microbes on advancing fat storage in the mammalian host. Although some bacteria are associated with excess adiposity, others have been directly implicated in improving metabolic syndrome and atherosclerosis, such as Akkermansia muciniphila , and are often found to be underrepresented in obesity. Administration of A muciniphila during obesity was shown to improve glucose tolerance. Schneeberger and colleagues found that among 27 genes that regulate inflammation and metabolism in white adipose tissue under high-fat feeding, 20 genes negatively correlate with the relative abundance of A muciniphila . In addition, Bifidobacterium spp also negatively correlated with 6 of 27 genes. Positive correlations were observed with Bilophila wadsworthia in 14 of 27 genes, and this microbe is known to expand under high-milk-fat diets and subsequently stimulate inflammatory responses. Together these observations suggest that certain microbes might regulate aspects of peripheral metabolism related to obesity and metabolism, but further investigations to determine strong proof of causality are required.
Another line of evidence that supports the notion that microbes might closely regulate host metabolism and body weight is found through the study of acute malnutrition in childhood. In contrast with overfed and obese individuals, work by Subramanian and colleagues followed severely malnourished children for 2 years. Through compositional modeling, they showed that the microbiota normally develop with growing children, but, with malnutrition, the microbiota maturity remains stunted and lags behind host development. Even after common therapeutic food interventions, the immaturity of the microbiota persisted. Note that the microbiota remains immature even under less severe malnourished states and that microbiota maturity correlated with anthropometric measurements of the children. These findings strongly support the notion of the gut microbiota functioning as a vital organ, and its development and growth throughout life may have important unknown implications for human health. Understanding the role of microbial development under states of hyperalimentation may provide insights into dysfunctional microbial-host metabolic interactions that lead to excessive fat storage.
Dietary Impact on the Gut Microbiota
Although host phenotype influences the composition of the microbial communities, diet also notably has an immediate and dramatic impact on microbial structure that mimics communities seen in obese individuals. For instance, David and colleagues observed in humans that a diet rich in animal-derived fat and protein resulted in significant changes in the gut microbiota in as little as a day, and, of particular note, blooms in hydrogen sulfide–producing bacteria such as B wadsworthia were also observed. Later, his group found that diet has a greater impact on altering microbial assemblage than genetic background in mice. In this study, 5 different inbred mouse strains, 4 genetic knockout strains relevant to host-microbe interactions (eg, ob/ob, NOD2, MyD88−/−, and Rag1−/−), and 200 outbred mice were placed on high-fat, high-sugar diets or diets rich in plant polysaccharides. In each experiment, the Western diet had profoundly altered community structure, regardless of strain differences or gene deficiencies. More recently, Sonnenburg and colleagues showed that diets low in microbe-accessible carbohydrate (MAC) and high in simple sugars result in loss of bacterial diversity and extinction of specific microbial groups that is compounded over generations. The generational loss of bacterial diversity could only be remedied with FMT from control mice maintained on the MAC-rich diet, but not by diet alone. This study provides a model for the rapid and drastic impact of the human food supply, containing readily available processed high-fat and high-sugar food, on the progressive loss of bacterial diversity over the past several decades. This theory postulates that human bodies are not equipped to reciprocate and adapt to the sudden insult on the gut microbiota, thereby leading to the development of obesity. Based on the results from this study, suitable therapies to combat the loss of bacterial diversity might include probiotic supplementation or FMT.
High-fat diets also transform the metagenomes of the bacteriophage community, also known as the phageome. It was shown by Howe and colleagues that high-fat diets can shift the phageome independent of observed alterations in the bacterial host pattern. The impact of diet on viral communities was also rapid, occurring within 24 hours. In addition, the change in the viral metagenomes by the high-fat diet was not reversible after washout, suggesting that diet-mediated changes in the phage community are persistent, similar to the aforementioned findings in bacteria. Further research in this area is needed to better understand the regulation and function of the phageome, its impact on gut microbial ecology, and more importantly consequences for the host.
Restructuring of Gut Microbiota in Bariatric Surgery
Surgical intervention, although largely invasive, is the most effective weight loss strategy for obesity. RYGB is the most common and the most effective, promoting a 20% to 40% weight loss compared with 15% to 30% loss of body weight with gastric banding. RYGB includes the formation of a small pouch, made of the upper stomach, that is then attached to a region of jejunum approximately 75 cm distal to the stomach (termed a gastrojejunostomy). The resulting limb (including the distal stomach) carries bile, gastric juice, and pancreatic juices alone, without nutrients, another 125 cm distal from the gastrojejunostomy, collectively delaying the mixture of digestive juices and nutrients for approximately 200 cm of the upper gastrointestinal (GI) tract. It is becoming increasingly apparent that bariatric surgery, particularly RYGB, may involve multiple mechanisms beyond simple physical restrictions to nutrient intake and absorption through reduced stomach size and decreased absorptive capacity. It is plausible that new treatments will be discovered based on the mechanisms underlying bariatric surgery efficaciousness. Intriguing data suggest that the mechanisms involved may include altered gut microbial function and interactions of the microbiome with the host’s bile acid pool.
Anatomic rearrangement triggers the dramatic restructuring of the intestinal microbiota and host-microbe interactions that may contribute to weight loss after bariatric surgery. For example, RYGB in mice resulted in the rapid restructuring of gut microbiota as early as 1 week compared with sham controls. The early changes in microbial composition occur within the same time frames as improvement in glucose tolerance and reduced insulin resistance, in contrast with body weight and adiposity changes that occur over weeks and months, suggesting that microbial alterations may be involved in the resetting of metabolic set points that are distinct from adiposity. Specifically, RYGB results in a decrease in the Firmicutes/Bacteroidetes ratio, which includes increases in Bacteroidales, Enterobacteriales, as well as increases in Gammaproteobacteria ( Escherichia coli ) and Verrucomicrobia as a relative percentage of the microbial community. Note that the Verrucomicrobia genus Akkermansia uses host secretion of mucin as a fuel source and has been inversely correlated with body weight. As previously discussed, oral administration of live A muciniphila restores insulin sensitivity in high-fat fed animals. Following RYGB in diabetic rodents, the level of A muciniphila in the small bowel increased significantly compared with sham obese controls. The increase in A muciniphila was positively related with the release of GLP-1, an important intestinal incretin, suggesting that this microbe could be modulating peripheral glucose handling through modulated insulin tolerance. In humans following RYGB, an inverse correlation in the relative percentages of E coli , Bacteroides , and Prevotella with circulating leptin levels was found, an important adipose factor released at higher levels in obesity. In addition, the increases in Proteobacteria observed following RYGB have reached 50-fold, and together with other models suggest that Proteobacteria may influence insulin sensitivity. A direct role for the microbial community in mediating host metabolism following RYGB was confirmed with FMT from bariatric surgery donors into recipients, which conferred protection from obesity. Altogether, these studies provide strong evidence that the gut microbiota may significantly contribute to the effectiveness of RYGB surgery, paving the way for focused investigations into altering the microbiota in a similar manner for the treatment of obesity.
The direct role for microbes in improving metabolism following RYGB remains under investigation, but other indirect roles include microbial changes to the bile acid composition; bile acid activation of the ileal and colonic bile acid receptors; and regulation of gut peptide enteroendocrine hormones, such as GLP-1 and peptide tyrosine tyrosine (PYY). Current evidence suggests that the composition of bile acids influences the microbiota assemblage through antimicrobial function, because bile acids are detergents and influence the membrane chemistry. Reciprocally, bacteria influence bile acid composition by deconjugation and fermentation of primary bile acids into secondary and tertiary bile acids, which have differential effects on host metabolism. Primary bile acids are associated with improved metabolism, whereas secondary bile acids are potentially carcinogenic and not associated with metabolic improvement. Therefore, bile acids and microbial compositions are inseparably associated and continually interacting in the gut. Novel work showed that bile acid–altered microbial communities in turn influence host metabolism, establishing a crosstalk between bile acids and the intestinal microbiome that influences host metabolism. In addition to altering bacterial viability and growth, and aiding in the absorption of luminal dietary lipids and lipid vitamins, bile acids directly modulate host metabolism through host bile acid receptors. Bile acid interactions with the G protein–coupled bile acid receptor 1 (GPBAR1 or TGR5) and farnesoid X receptor (FXR) regulate peripheral energy expenditure and counteract obesity and diabetes. On activation by bile acids, TGR5 specifically stimulates the release of GLP-1, GLP-2, and PYY from enteroendocrine cells as well as expression of various transport proteins and biosynthetics, resulting in improved glycemic control. Enteroendocrine cells also contain toll-like receptors and sense bacteria in the intestinal lumen. Following RYGB, increased circulating levels of GLP-1 and PYY are reported. PYY is normally released postprandially to increase energy expenditure and decrease food intake. In healthy individuals, PYY release following feeding is proportionate to caloric consumption, acting directly on the hypothalamus and vagal afferents to slow feeding behavior. It remains unclear whether increased PYY and GLP-1 levels following RYGB are in response to altered microbial populations, bile acid pools, or a combination. Regardless, changes in intestinal enteroendocrine signaling following RYGB have profound effects on host metabolism and multiple lines of evidence now strongly support the involvement of the microbiota.
Host-microbe interactions driving obesity
The observation that GF mice are resistant to diet-induced obesity has created a foundation for understanding the contribution of microbes and host-microbe interactions to the development of obesity and its comorbidities. Several mechanisms to explain microbe-mediated obesity have been proposed, including (1) SCFA production; (2) regulation of food intake and sensory perception of food; (3) nutrient absorption; (4) circulation of microbe-derived enterotoxins like lipopolysaccharides (LPS) and reduced production of angiopoietinlike 4 (angptl4), resulting in increased fatty acid uptake in liver and adipose tissue ; and (5) peripheral control of circadian rhythm, which is intimately linked to metabolic coordination between the brain and peripheral organs.
One metabolic function of microbes is the production of SCFAs, including acetate, propionate, and butyrate, from otherwise indigestible fibers. SCFAs can act as energy sources for the intestinal epithelium and liver, and mouse models of obesity show increased SCFA in luminal content and lower energy content in feces. However, SCFAs have many reported beneficial effects on metabolism and improved glucose tolerance. For instance, diets supplemented with fructo-oligosaccharides (FOSs), butyrate, and propionate decreased weight gain and improved glucose tolerance in rats compared with controls. It was shown that these positive effects were mediated through stimulation of intestinal gluconeogenesis, because mice deficient in the catalytic subunit of glucose-6 phosphatase displayed impaired glucose tolerance. Thus, conflicting evidence exists regarding the negative consequences of increased energy availability through SCFA production given the potential positive impact of SCFAs on metabolism.
Obesity is also related to peripheral inflammation, especially in adipose tissue. The gut microbiota influences gut permeability, which may lead to entry of microbial ligands, including LPS, into the blood stream and periphery, where they can induce insulin resistance and prevent peripheral uptake of fat. Intriguingly, bioactive dietary components such as omega 3 fatty acids and polyphenols that are reported to improve adipose inflammation also affect microbial structure. For example, Backhed’s group showed that the gut microbiota exacerbates adipose inflammation through toll-like receptor signaling on saturated fat feeding, as has been suspected for some time in adipose biology. Notably, microbiota transplant from fish oil–fed mice attenuated weight gain in antibiotic mice that were maintained on a lard diet. Cranberry and grape polyphenols have also been reported to alter microbial structure, specifically via increasing the abundance of A muciniphila , as well as improving glucose tolerance and adipose inflammation.
Microbial regulation of metabolism is mediated in part through the sensory perception of food, GI motility, and nutrient absorption, because these are altered in GF mice. For instance, GF mice have increased preference for sugar-sweetened liquids and fat emulsions but lack the machinery to process the nutrients. Swartz and colleagues found that GF mice consume more sucrose solution concurrent with increased expression of type 1 taste receptor 3 (TIR3) expression and sodium glucose luminal transporter 1 (SGLT1) in the small intestinal epithelium compared with conventional mice. Anorexigenic gut peptide hormones, including PYY and cholesytokinin (CCK), are also regulated by gut microbes facilitating control of food intake. Although GF mice have increased expression of lingual fatty acid translocase (CD36), expression of gut peptide hormones, including PYY, CCK, and GLP-1, was reduced in the intestinal epithelium, as well as decreased numbers of enteroendocrine cells (EECs) in the ileum. In contrast with these gut peptide hormones, Backhed’s group reported that GF mice have increased levels of GLP-1, which slows gastric motility and increases intestinal transit time as a compensatory mechanism to allow enhanced nutrient absorption. Given the role of enteroendocrine hormone signaling in nutrient absorption, dysregulation of these hormones may explain why GF mice have increased levels of triglycerides and total lipids in their stool after high-fat diet feeding. In addition, conventionalization of GF zebrafish increases lipid accumulation in the intestinal epithelium. Taken together, these findings suggest that gut microbes facilitate hormonal cues to regulate sensory perception of food, dietary intake, as well as nutrient absorption. However, the exact mechanisms behind microbial regulation of carbohydrate and lipid absorption and the extent to which microbe-induced nutrient absorption significantly contributes to obesity have not been well characterized.
Differences between GF and conventional mice also involve dysregulation of bile production. Because of the lack of microbes in GF mice, there is little to no deconjugation of conjugated bile acids entering the GI lumen, thereby resulting in high levels of taurine-conjugated bile acids in GF mice compared with conventional mice. Backhed’s group reported that increased taurine-conjugated bile acids block FXR-mediated induction of fibroblast growth factor 15 (FGF-15), which would otherwise decrease bile acid synthesis in the liver. Thus, GF mice have increased bile acid production. This study implicates the role of gut microbiota in regulating bile acid metabolism through a gut-liver axis.
The difference in bile acid metabolism speaks to the marked difference in liver function between GF and conventional mice. GF mice have decreased liver lipid content and altered expression of gene networks, including those involving xenobiotic metabolism and circadian rhythm. At the hub of xenobiotic gene networks are 2 nuclear hormone receptors, constitutive androstane receptor (CAR) and pregnane X receptor (PXR), which are implicated in regulating whole-body metabolism. Activation of CAR has been shown to decrease body weight and improve insulin sensitivity, whereas PXR activation has been positively associated with obesity. Thus, it is tempting to speculate that CAR-mediated metabolic activity in GF mice may contribute to their resistance to high-fat diet–induced obesity. However, this connection has not been thoroughly investigated in the current literature.
Gut microbes have been found to control circadian function. This finding has important implications for fighting obesity, because the disruption of the natural cycle of day and night (eg, jet lag, shift work, and sleep apnea) contributes to the increasing prevalence of metabolic disorders. Circadian rhythms are regulated by molecular clocks that coordinate regularly timed events (ie, states of feeding vs fasting) and the necessary physiologic responses to enhance metabolic efficiency. Thus, circadian rhythm is intimately linked to the regulation of food intake, activity, and whole-body metabolism involving clocks located in the brain as well as peripheral metabolic tissues. The circadian transcriptional program is under the control of 2 major transcriptional activators, Bmal and Clock, which are counter-regulated by repressors, Period 1 to 3 and Cryptochrome 1 and 2. Consumption of high-fat diets represses diurnal variation of these gene transcripts and impairs normal circadian function. It has recently been shown that these changes depend on the gut microbiota, because GF mice and antibiotic-treated mice have reduced expression of Bmal and Clock and increased expression of Period 1 to 3, and Cryptochrome 1 and 2 in the intestinal epithelium. It was later shown by Leone and colleagues that diurnal variation in the circadian gene program is also blunted in the liver of GF compared with specific pathogen free (SPF) mice.
In addition to host circadian rhythm, microbes display circadian behavior. Strikingly, community structure of the gut microbiota as well as butyrate shows diurnal variation over a 24-hour period under normal feeding conditions and is diminished under high-fat feeding. To ensure that these changes were not caused by times of feeding, stool was collected from mice on total parenteral nutrition (TPN) and compared with mice fed enterally. Although differences existed in the relative abundance of specific microbes (eg, increase in Verrucomicrobia in the TPN group), diurnal shifts were still evident, indicating that microbial abundance may fluctuate based on host cues, such as the release of mucin or other epithelial proteins and secretions. Altogether these findings suggest that the regulation of host circadian function depends on the activity of the gut microbiota and, conversely, the circadian behavior of the gut microbiota depends on host physiology. Identifying the host-microbe interactions that facilitate microbial control of circadian rhythm may lead to therapies targeting the gut microbiota to restore the metabolic consequences of disrupted sleep, which is common in obesity.
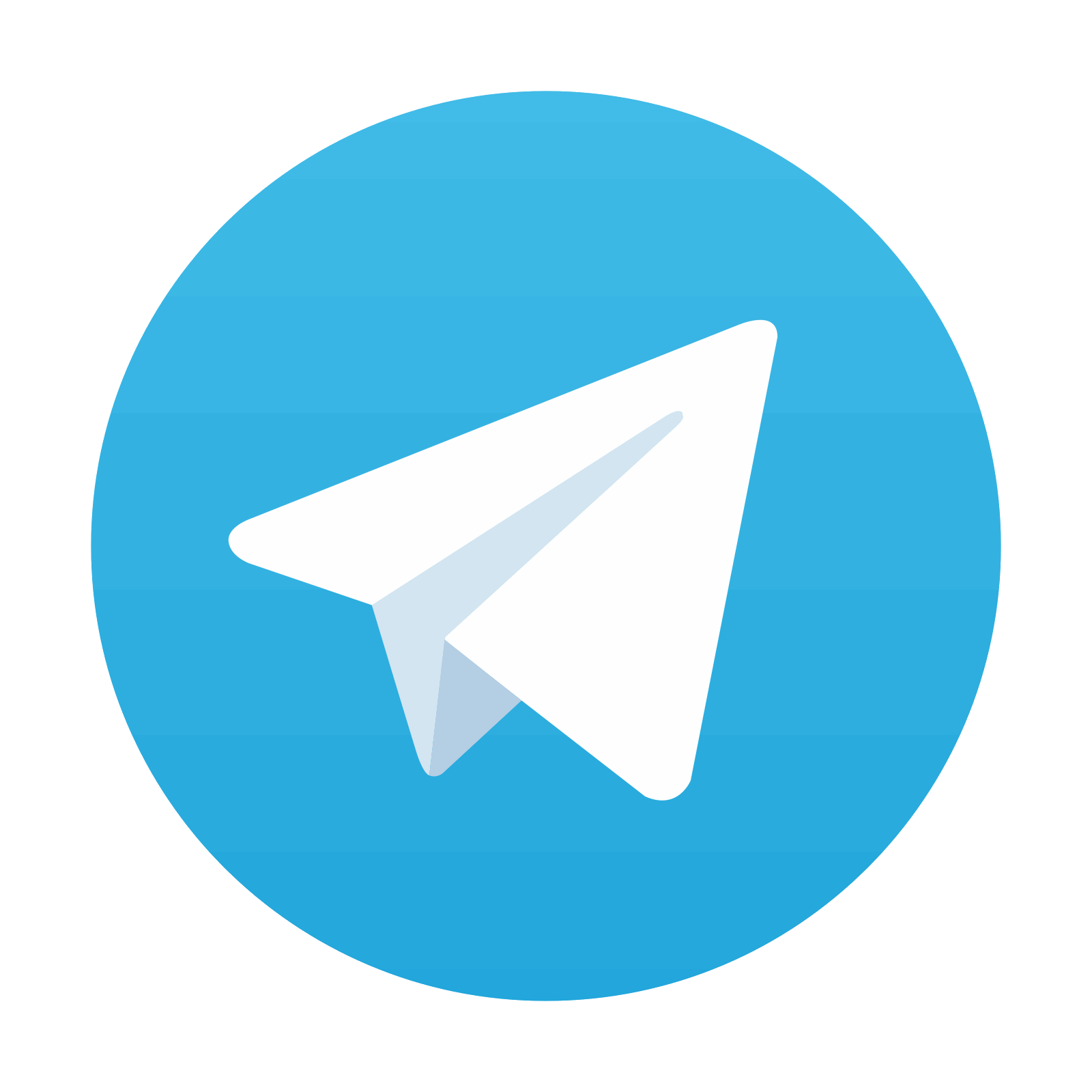
Stay updated, free articles. Join our Telegram channel

Full access? Get Clinical Tree
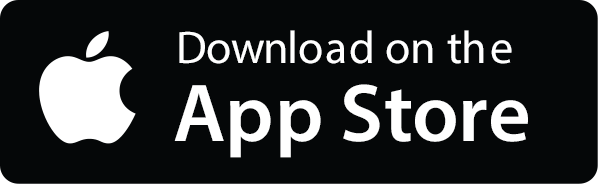
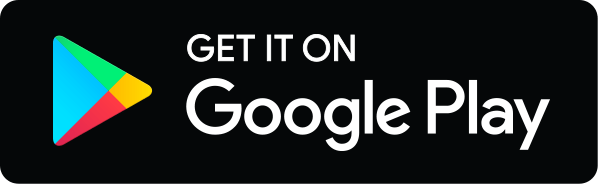